Vision
With far more than 100 million nerve cells, the retina is the first stage of our visual system and our window to the outside world. The process includes detection of light impulses (photons) by different light receptors, in general called rods (120 million cells) and cones (6 million cells), and the fast and continuous translation, filtration and post-procession into electrical signals (or nerve impulses). These signals are then passed through the optical nerve’s 1.5 million fibres to the visual centre of the brain and reinterpreted into a cohesive image. The flexibility and economy with which retinal cells work together remains beyond our powers of imagination.
This series of images is showing the invisibly small, yet perfectly shaped structures that are essential parts of our predominant sensory organ – the eye. The eye-project compares the human eye to similar ‹visual tools› that evolved in other creatures. Often the design of such structures is very sophisticated and sensationally beautiful. Some of these structures may one day inspire scientists and engineers to develop innovative tools for new microscopic investigations into the unseen… (e.g. minimal-invasive-surgery, micro-technology, digital photography, astonomy, etc).
«The print looks beautiful! (…) It really is extraordinary work… you are a modern master!»
Bill Hearl, PhD
CEO Immunomic Therapeutics, Inc.
Micronaut images are rights-managed. If you want to get a quote, please contact us, providing the following information: (1) image name, (2) specific use, (3) industry, (4) distribution area, (5) format, (6) circulation or print run, and (7) duration.
Please note that we cannot answer incomplete requests. Thank you.
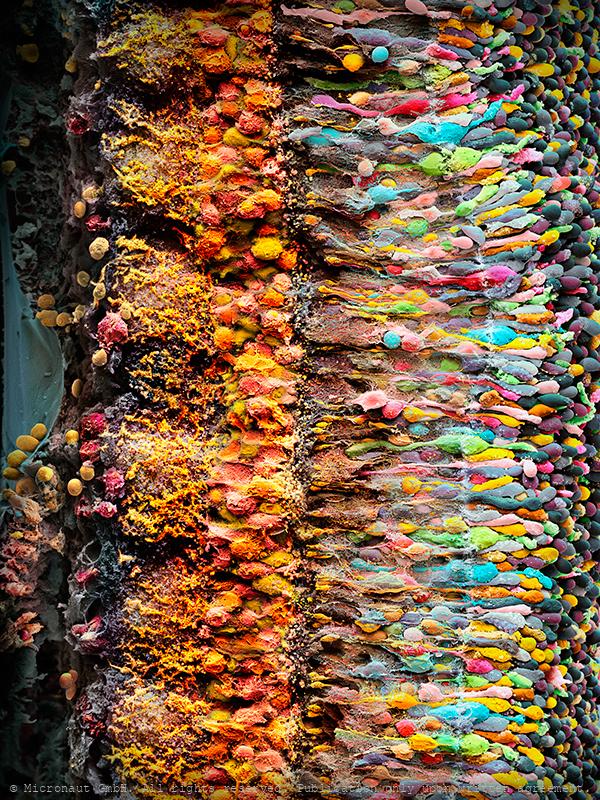
The human Retina - Nr. 2 (2019), vertical
With far more than 100 million nerve cells, the retina is the first stage of our visual system and our window to the outside world. The process includes detection of light impulses (photons) by different light receptors, in general called rods (120 million cells) and cones (6 million cells), and the fast and continuous translation, filtration and post-procession into electrical signals (or nerve impulses). These signals are then passed through the optical nerve’s 1.5 million fibres to the visual centre of the brain and reinterpreted into a cohesive image.The flexibility and economy with which retinal cells work together remains beyond our powers of imagination: the eye reports numerous signals to the brain at once, including separate detection of light (light on) and dark (light off), general patterns and finest details, movements and different hues (including: red, green and blue). This literally makes the eye a camera with 10 to 15 different films and despite it appears so naturally to us, the perception of an image is the result of a most complex interaction process involving millions of cells and the electrical signals they produce and pass on to the brain. What you can see on this image is the (1) rods and cones layer. It is located below the pigment epithelia which has been cut away during the preparation and is not visible on this image (black space on top). Below the rods and cones layer you can find the (2) outer nuclear layer (ONL) which contains the nuclei of the rods and cones. The adjacent (3) outer plexiform layer (OPL) is followed by the (4) inner plexiform layer (IPL), which contains numerous cell types, including horizontal cells, bipolar cells and amacrine cells. At the very bottom you can see the (5) inner nuclear layer (INL), followed by the (6) ganglion cell layer with two (7) blood vessels shown at left and (8) nerve fibers (which form the optical nerve).
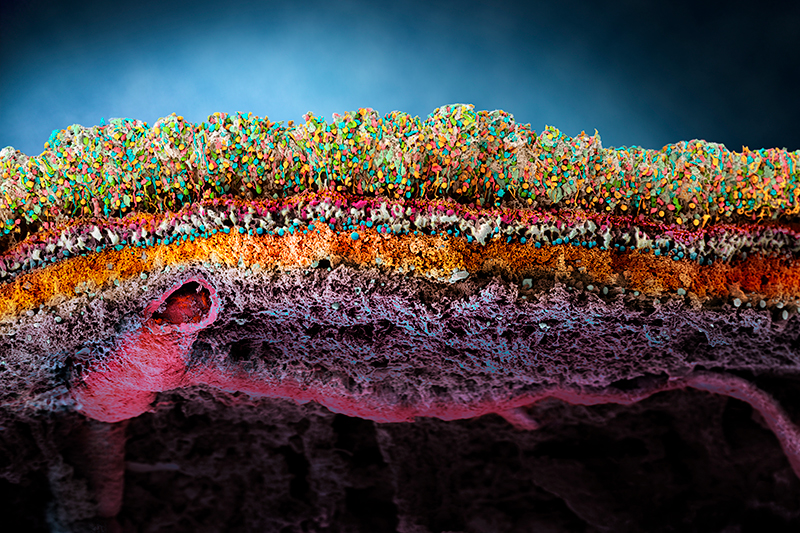
Porcine retina (Sus domesticus)
Porcine Retina (Sus domesticus) With far more than 100 million nerve cells, the retina is our first stage of the visual system and window to the outside world. The picture highlights the neuronal layers of a porcine retina. Seungkuk Ahn and his colleagues have electromagnetically guided viruses to specifically transduce each of the retina layers. The process inside the retina includes detection of light impulses (photons) by different light receptors, in general called rods (120 million cells) and cones (6 million cells), and the fast and continuous translation, filtration and post-procession into electrical signals (or nerve impulses). These signals are then passed through the optical nerve’s 1.5 million fibres to the visual centre of the brain and reinterpreted into a cohesive image.The flexibility and economy with which retinal cells work together remains beyond our powers of imagination: the eye reports numerous signals to the brain at once, including separate detection of light (light on) and dark (light off), general patterns and finest details, movements and different hues (including: red, green and blue). This literally makes the eye a camera with 10 to 15 different films and despite it appears so naturally to us, the perception of an image is the result of a most complex interaction process involving millions of cells and the electrical signals they produce and pass on to the brain.
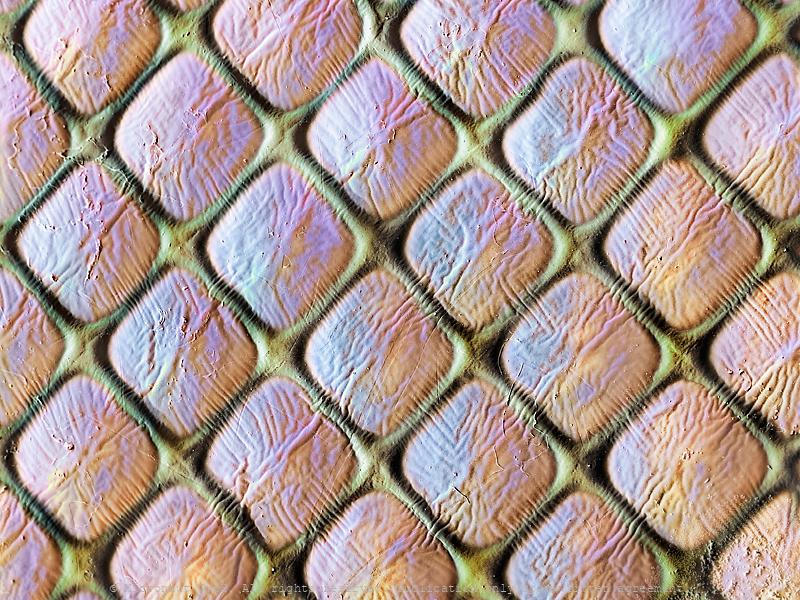
Evolution of Eyes (Mirror-Box)
Sunlight was and still is one of the most important selective forces for the evolution of living organisms. As a consequence, catching photons and obtain high quality vision is under strong selection pressure which has lead to the evolution of many different types of eyes under various environmental conditions, over the last 5 billion years. In this image the surface (lens) area of a crustacean compound eye is shown. In general, each unit, or ommatidium, consists of a lens that forms an image onto a small number of receptor cells (which are called 'rhabdom') and the resolving power of compound eyes depends on the size and number of facets. In contrast to insects, whatsoever, shrimps and lobsters have compound eyes with square, homogeneous box-like structures. This has caused considerable confusion in the 1950s and 1960s because no optical function could be ascribed to square lens elements and for more than 20 years shrimps were believed to be blind. However, in these animals ray-bending is not done by the lens but by mirror in the wall of each omatidia and the mirror-box design only works with right-angle corners and not hexagons, which accounts for the square facets. Clearly, the resultant image as seen by insects and crustaceans is of comparable quality.
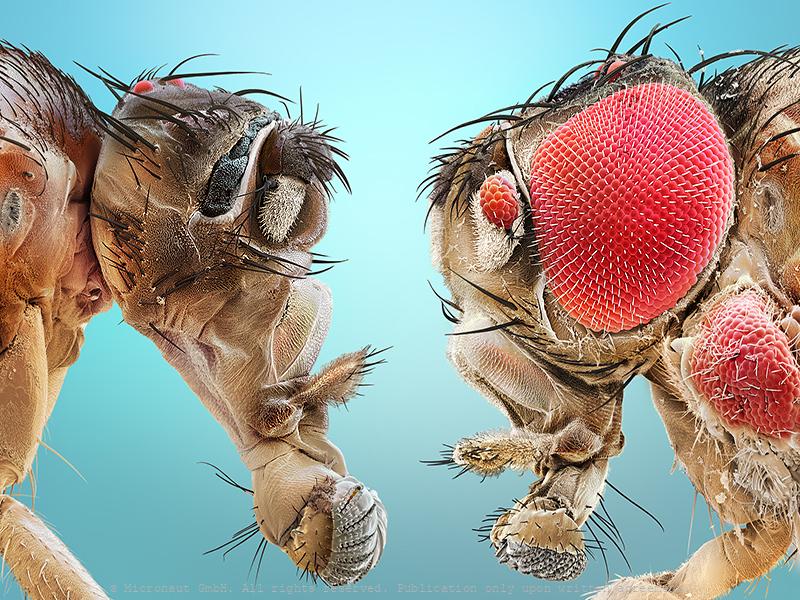
Uneven brothers (D. melanogaster), Nr.3
Coloured scanning electron micrograph (SEM) of two mutant Fruitfly (Drosophila melanogaster) heads. Wildtype (i.e. normal) Fruitflies have two compound eyes (red) - one on either side of the head. Small bristles between the single lenses of the eye make sure it cannot be covered with dust or dirt particles. Genetically manipulated Flies can either lack compound eyes completely (left) or have additional eyes on the antennae, legs and other body areas (right). Fruit flies are widely used in genetic experiments, particularly in mutation experiments, because they reproduce rapidly and their genetic systems are well understood. This image visualizes how easily the results of the genetic modification can be observed in the Fruitfly which is one of the main reasons why it is still the most frequently used model organism in genetics despite more than 100 years of experimental research. left: Sine oculis-1 (so1) variant which lacks the compound eyes completely. right: Eyeless variant with ectopically expressed compound eyes under dpp-promotor in all imaginal discs. Handkoloriertes Raster-Elektronen-Mikroskopiebild zweier Fruchtfliegen-Mutanten. Die Taufliege oder Fruchtfliege (Drosophila melanogaster) ist das klassische Untersuchungsobjekt in der Genetik und Entwicklungsbiologie. Wildtypen, d.h. genetisch unveränderte Fruchtfliegen, besitzen zwei grosse rote Komplexaugen - je eines auf jeder Seite des Kopfes. Die Komplexaugen werden durch Borsten zwischen den winzigen Einzelaugen vor Verschmutzung und Staub geschützt. Die Entwicklung des Auges wird während der Embryonalentwicklung Larve durch bestimmte Gene gesteuert. Durch Veränderungen (Mutation) von Genen lassen sich z.B. Position oder Morphologie der Komplexaugen gezielte beeinflussen. Im Vergleich zu einem Säugetier ist die Fruchtfliege genetisch viel einfacher aufgebaut und weist lediglich vier Paar Chromosomen auf. Diese Tatsache hat, neben der raschen Generationsfolge und einfachen Zucht, wesentlich dazu
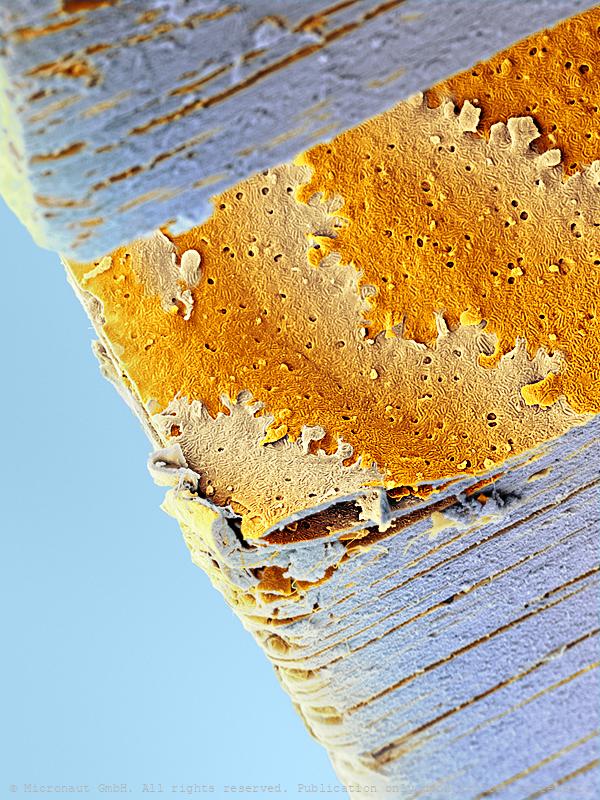
Human Lens cross-section, Nr.2
Cross-section of a human lens of a human eye. It is the only transparent tissue in the human body. In the past few years, scientists have determined that this transparency —critical for focusing light— stems in large part from the unique ability of the lens to acti- vate a self-destruct program in its cells that aborts just before com- pletion, leaving empty but sustain- able cells that transmit visible rays. Layers of lens cells align in parallel (top and bottom), so that light passes perpendicularly through them. Within a layer (center), adjacent cells interlock like jigsaw puzzle pieces to prevent gaps from forming when the lens changes shape during focusing; the layering and interlocking of cells enables light to pass across cell boundaries without scattering.
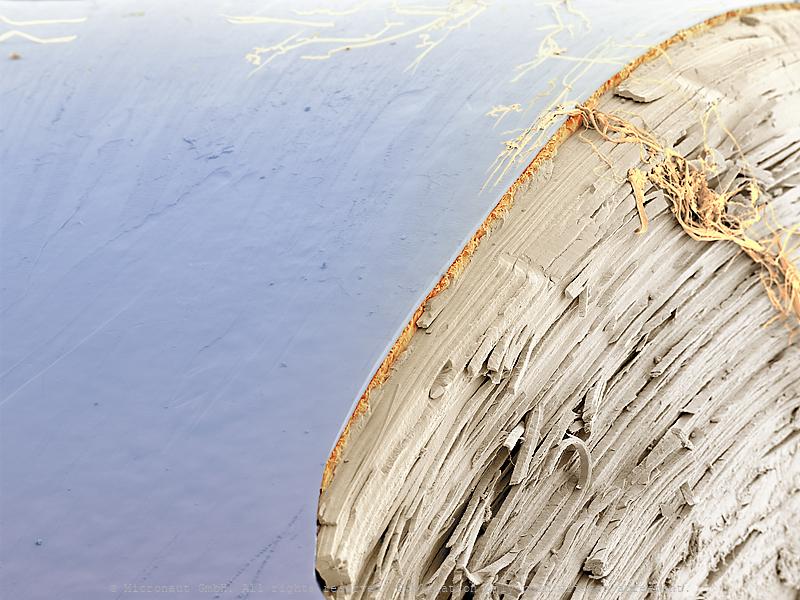
Human Lens cross-section, Nr.1
Cross-section of a human lens of a human eye. It is the only transparent tissue in the hu- man body. In the past few years, scientists have determined that this transparency —critical for focusing light— stems in large part from the unique ability of the lens to acti- vate a self-destruct program in its cells that aborts just before com- pletion, leaving empty but sustain- able cells that transmit visible rays. Studies of the lens of the eye not only could reveal ways to prevent cataracts but also might illuminate the biology of Alzheimer’s, Parkinson’s and other diseasesin which cells commit suicide.
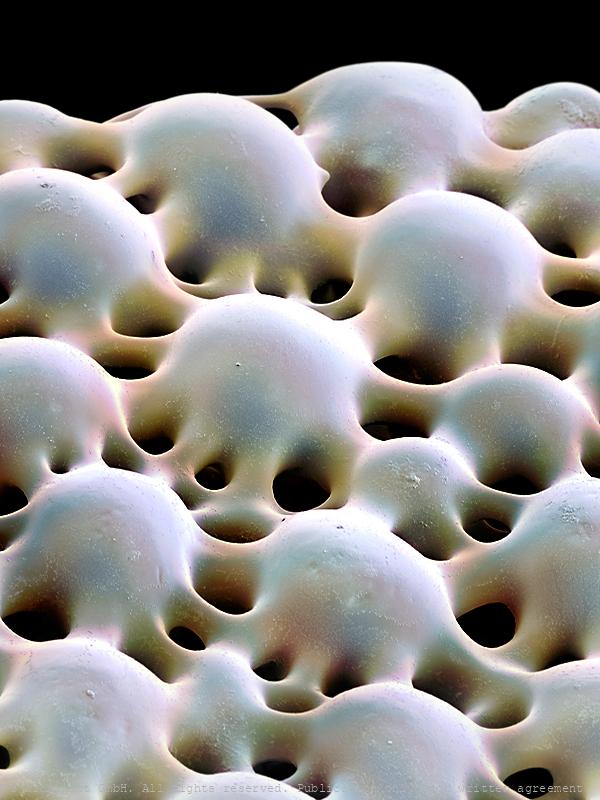
Evolution of Eyes (Calcitic Microlens), Nr.1
"In a clever twist of nature, the sea has eyes in its stars." John Roach, National Geographic. Scientists have discovered a species of brittle star, Ophiocoma wendtii, with crystalline microlens plates in its skeleton that have exceptional optical performance: according to Gordon Hendler and Joanna Aizenberg, the scientists that made the discovery, the microlens plates in the brittle stars outer skeleton are even compensated for physical effects that bother us when we fabricate lenses in the laboratory (birefringence and spherical aberration). From the microlenses the light is focused onto nerve bundles located beneath which transmit the optical information to the rest of the body. Although yet unproven, the whole photoreceptive system of the brittle star is thought to function like a compound eye, allowing the detection of predators or seeking out hiding places. The study of creatures such as O. wendtii has important implications for science. How the brittle star's visual system works may be useful, for example, in developing technologies for chip design in optical networks and better lithographic techniques. Thereby, the microlens arrays of the brittle star O. wendtii proofs that nature is often a step ahead of people and foreshadows technical developments.
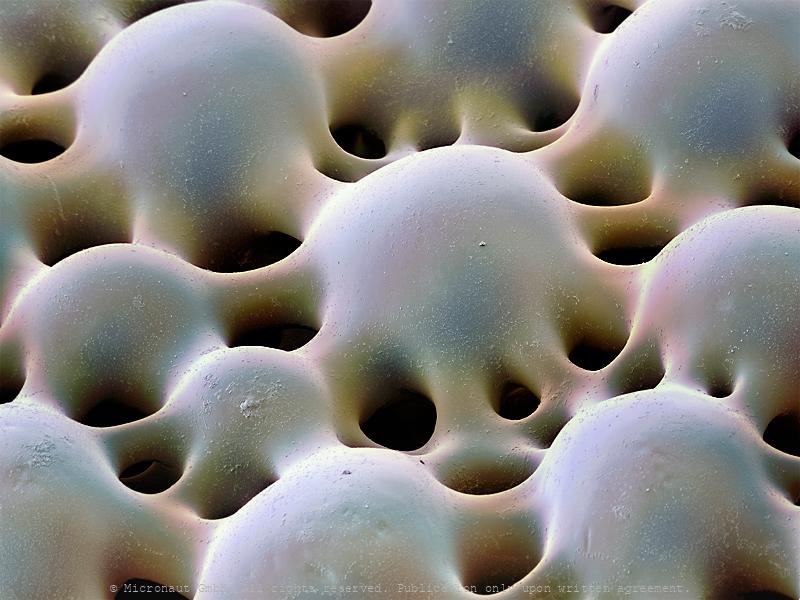
Evolution of Eyes (Calcitic Microlens), Nr.2
"In a clever twist of nature, the sea has eyes in its stars." John Roach, National Geographic. Scientists have discovered a species of brittle star, Ophiocoma wendtii, with crystalline microlens plates in its skeleton that have exceptional optical performance: according to Gordon Hendler and Joanna Aizenberg, the scientists that made the discovery, the microlens plates in the brittle stars outer skeleton are even compensated for physical effects that bother us when we fabricate lenses in the laboratory (birefringence and spherical aberration). From the microlenses the light is focused onto nerve bundles located beneath which transmit the optical information to the rest of the body. Although yet unproven, the whole photoreceptive system of the brittle star is thought to function like a compound eye, allowing the detection of predators or seeking out hiding places. The study of creatures such as O. wendtii has important implications for science. How the brittle star's visual system works may be useful, for example, in developing technologies for chip design in optical networks and better lithographic techniques. Thereby, the microlens arrays of the brittle star O. wendtii proofs that nature is often a step ahead of people and foreshadows technical developments.
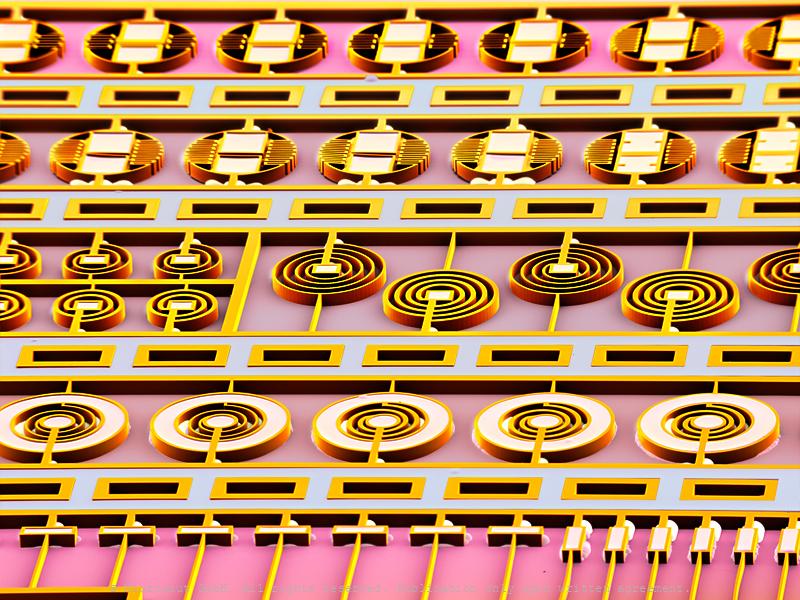
Micro Robots
Colored SEM image showing micro robots named 'PolyMites', arranged on a waver plate. They are the next generation of MagMites (magnetic mite robots). The PolyMites are not released from silicon wafer yet. In the end the robots will have a round shape without the two bars which hold each robot in place on the waver plate.
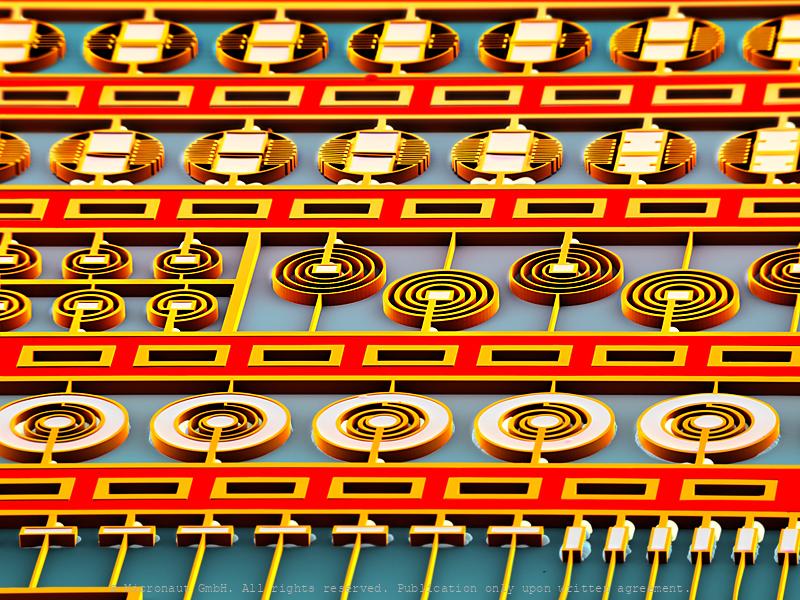
Micro Robots
Colored SEM image showing micro robots named 'PolyMites', arranged on a waver plate. They are the next generation of MagMites (magnetic mite robots). The PolyMites are not released from silicon wafer yet. In the end the robots will have a round shape without the two bars which hold each robot in place on the waver plate.
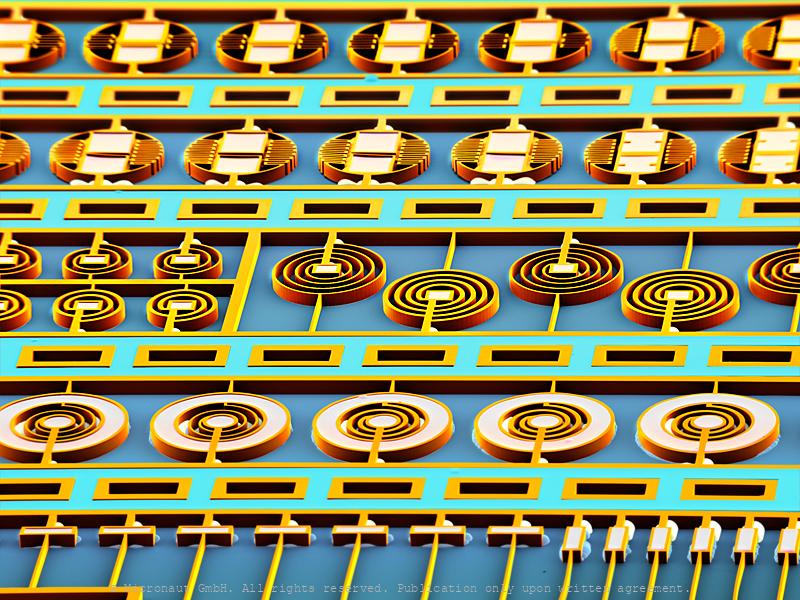
Micro Robots
Colored SEM image showing micro robots named 'PolyMites', arranged on a waver plate. They are the next generation of MagMites (magnetic mite robots). The PolyMites are not released from silicon wafer yet. In the end the robots will have a round shape without the two bars which hold each robot in place on the waver plate.
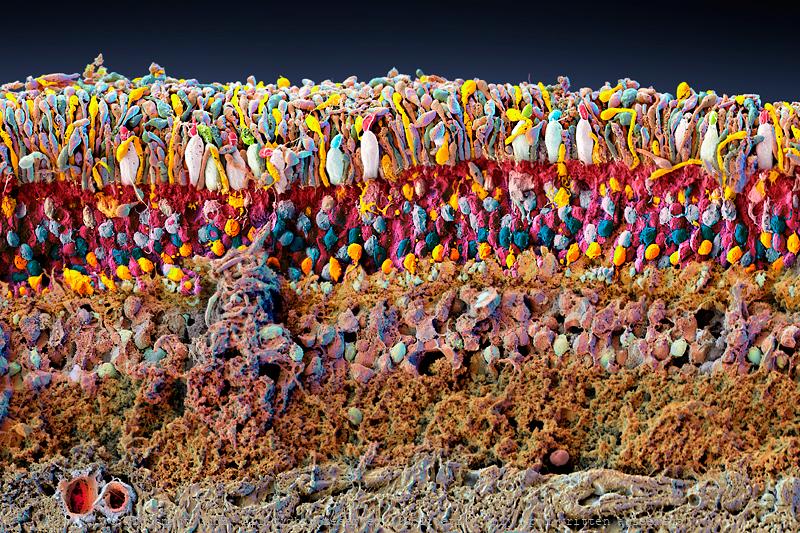
Beyond our imagination - the retina (of the human eye)
With far more than 100 million nerve cells, the retina is the first stage of our visual system and our window to the outside world. The process includes detection of light impulses (photons) by different light receptors, in general called rods (120 million cells) and cones (6 million cells), and the fast and continuous translation, filtration and post-procession into electrical signals (or nerve impulses). These signals are then passed through the optical nerve’s 1.5 million fibres to the visual centre of the brain and reinterpreted into a cohesive image.The flexibility and economy with which retinal cells work together remains beyond our powers of imagination: the eye reports numerous signals to the brain at once, including separate detection of light (light on) and dark (light off), general patterns and finest details, movements and different hues (including: red, green and blue). This literally makes the eye a camera with 10 to 15 different films and despite it appears so naturally to us, the perception of an image is the result of a most complex interaction process involving millions of cells and the electrical signals they produce and pass on to the brain. What you can see on this image is the (1) rods and cones layer. It is located below the pigment epithelia which has been cut away during the preparation and is not visible on this image (black space on top). Below the rods and cones layer you can find the (2) outer nuclear layer (ONL) which contains the nuclei of the rods and cones. The adjacent (3) outer plexiform layer (OPL) is followed by the (4) inner plexiform layer (IPL), which contains numerous cell types, including horizontal cells, bipolar cells and amacrine cells. At the very bottom you can see the (5) inner nuclear layer (INL), followed by the (6) ganglion cell layer with two (7) blood vessels shown at left and (8) nerve fibers (which form the optical nerve).
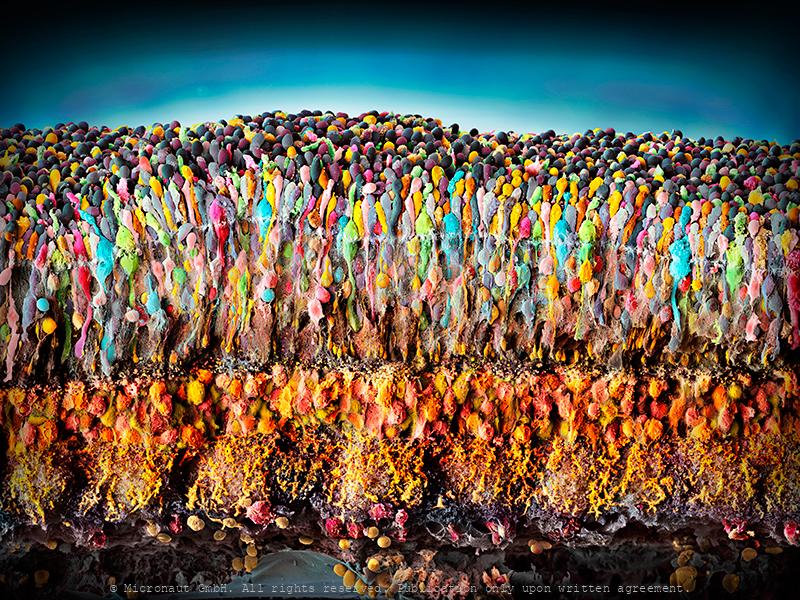
The human Retina - Nr. 2 (2019), horizontal
With far more than 100 million nerve cells, the retina is the first stage of our visual system and our window to the outside world. The process includes detection of light impulses (photons) by different light receptors, in general called rods (120 million cells) and cones (6 million cells), and the fast and continuous translation, filtration and post-procession into electrical signals (or nerve impulses). These signals are then passed through the optical nerve’s 1.5 million fibres to the visual centre of the brain and reinterpreted into a cohesive image.The flexibility and economy with which retinal cells work together remains beyond our powers of imagination: the eye reports numerous signals to the brain at once, including separate detection of light (light on) and dark (light off), general patterns and finest details, movements and different hues (including: red, green and blue). This literally makes the eye a camera with 10 to 15 different films and despite it appears so naturally to us, the perception of an image is the result of a most complex interaction process involving millions of cells and the electrical signals they produce and pass on to the brain. What you can see on this image is the (1) rods and cones layer. It is located below the pigment epithelia which has been cut away during the preparation and is not visible on this image (black space on top). Below the rods and cones layer you can find the (2) outer nuclear layer (ONL) which contains the nuclei of the rods and cones. The adjacent (3) outer plexiform layer (OPL) is followed by the (4) inner plexiform layer (IPL), which contains numerous cell types, including horizontal cells, bipolar cells and amacrine cells. At the very bottom you can see the (5) inner nuclear layer (INL), followed by the (6) ganglion cell layer with two (7) blood vessels shown at left and (8) nerve fibers (which form the optical nerve).
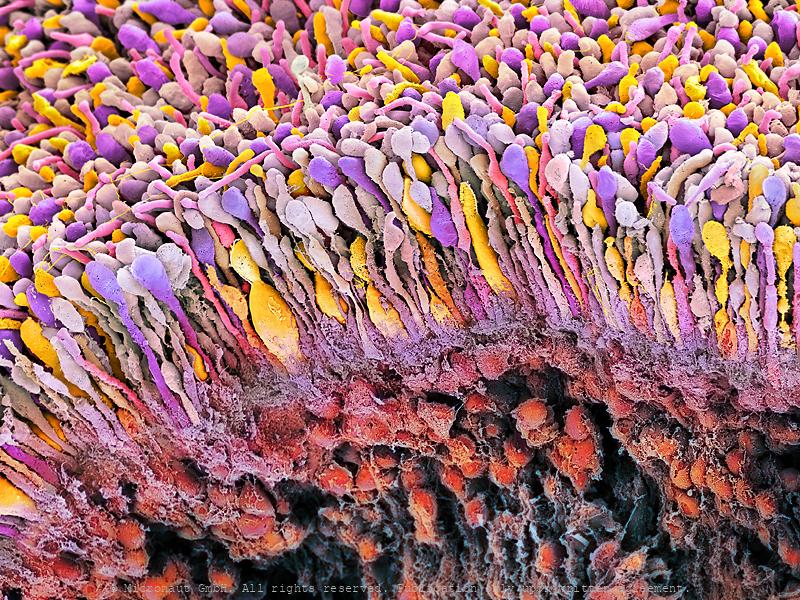
human Retina (Nr.3)
With far more than 100 million nerve cells, the retina is the first stage of our visual system and our window to the outside world. The process includes detection of light impulses (photons) by different light receptors, in general called rods (120 million cells) and cones (6 million cells), and the fast and continuous translation, filtration and post-procession into electrical signals (or nerve impulses). These signals are then passed through the optical nerve’s 1.5 million fibres to the visual centre of the brain and reinterpreted into a cohesive image.The flexibility and economy with which retinal cells work together remains beyond our powers of imagination: the eye reports numerous signals to the brain at once, including separate detection of light (light on) and dark (light off), general patterns and finest details, movements and different hues (including: red, green and blue). This literally makes the eye a camera with 10 to 15 different films and despite it appears so naturally to us, the perception of an image is the result of a most complex interaction process involving millions of cells and the electrical signals they produce and pass on to the brain.
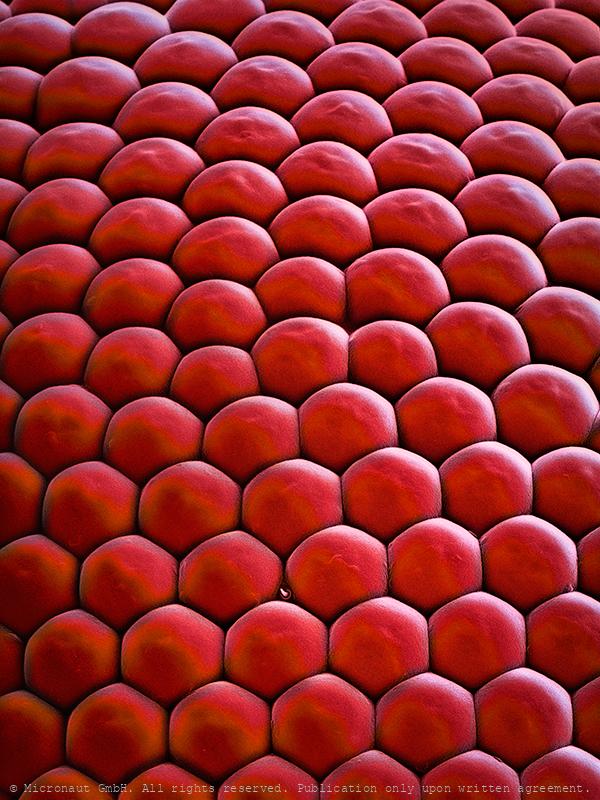
Compound eye
The eye of a moth consists of a tightly packed array with hundreds of small single lenses.
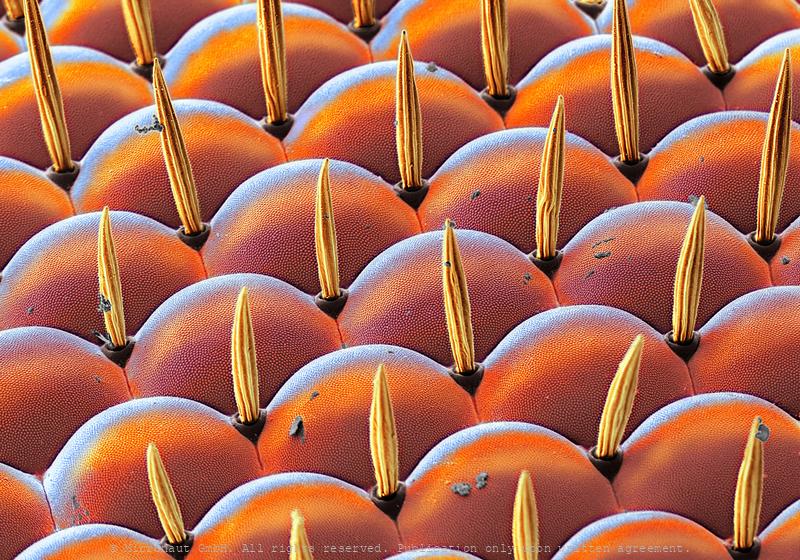
Compound eye (Drosophila melanogaster)
Coloured scanning electron micrograph (SEM) of a small area from the left eye from the common Fruitfly (Drosophila melanogaster). These small insects are widely used in genetic experiments, particularly in mutation experiments, because they reproduce rapidly and their genetic systems are well understood. Wildtype (i.e. normal) Fruitflies have two compound eyes (red) – one on each side of the head. Each eye is a regular structure built up with 750 repeating units, or single lenses. Each single lens sends signals to the fly’s brain where they are combined and arranged into a mosaic map of the visual “outside world”. Tiny bristles between the lenses make sure it cannot be covered with dust or dirt particles.
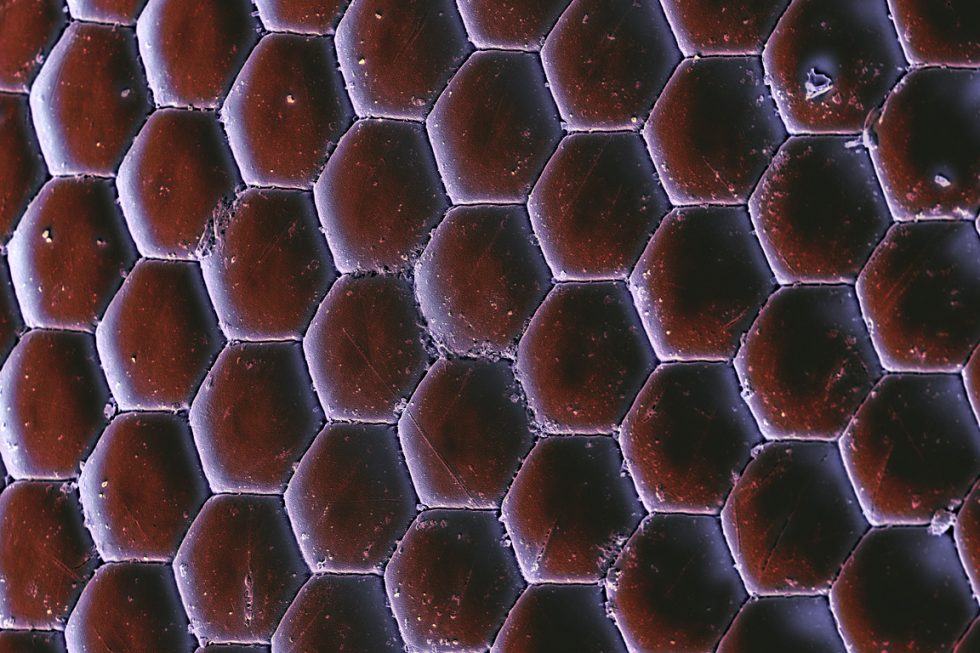
Jellowjacket compound eye (Vespa crabro)
Hornet compound eye
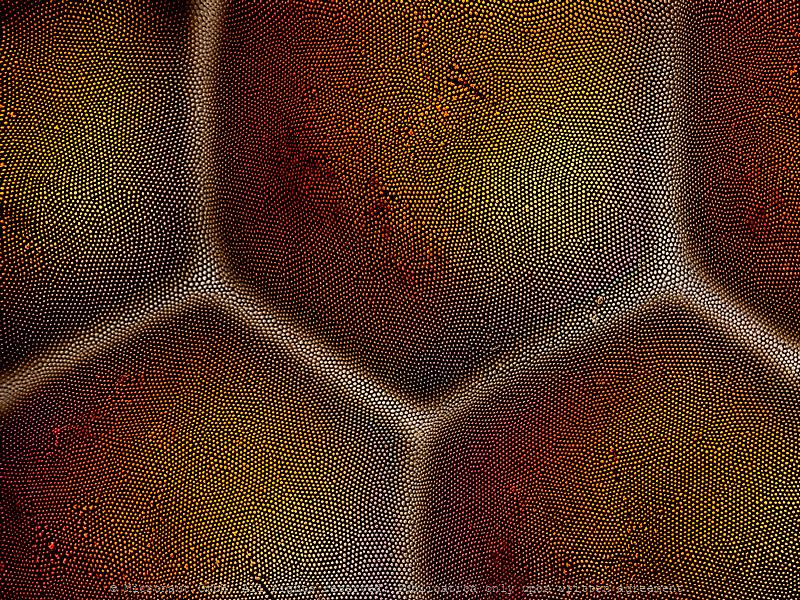
Anti-reflective coating, Nr.1
Antireflective Coating – ‘Corneal Nipple Arrays’ in Insect EyesEyes naturally possess a refracting surface - without it, image formation would be impossible. But if the transition in the refractive index is sharp, reflections might be produced which can impair image quality. Moreover, the bright glare from an animal's eye can alert predators. Therefore, some insects have evolved fascinating nanoscale anti-reflection devices in the surface of their compound eyes.The compound eye of the Old Lady (Mormo maura) moth reveals highly ordered nano-structured conical protrusions on the corneal cuticle. It has been shown that this so-called ‘corneal nipple array’ or ‘moth-eye array’ has almost perfect anti-reflection properties. The crucial factor to reduce reflection of light is the nipple height. Only nipples with a height of > 50-200nm are anti-reflective. Corneal nipple arrays best known from nocturnal moths and butterflies (Lepidoptera), however they are widespread among insects and have probably evolved more than once. The nipples of the Old Lady moth are arranged in a hexagonal, almost crystalline fashion. In parallel to their anti-reflective function, the nipples also increase the surface area and thereby enhance the light sensitivity of the moth, though light transmittance increase may only be of minor importance compared to the potentially life-saving ability of the anti-reflective coating.Studying nano-structured corneal nipple arrays of insect eye facets helps to design better amorphous silicon thin film solar cells and improves the antireflective coating on notebooks and smartphones.
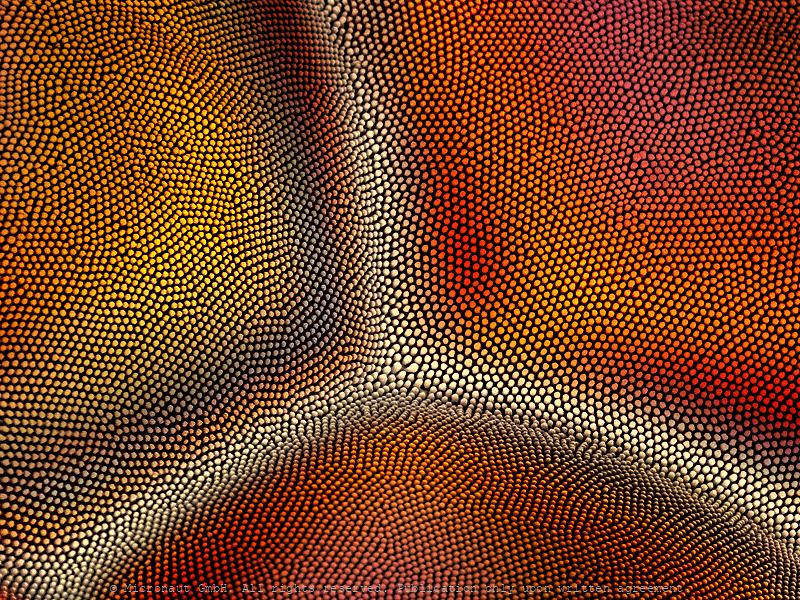
Anti-reflective coating, Nr.2
Antireflective Coating – ‘Corneal Nipple Arrays’ in Insect EyesEyes naturally possess a refracting surface - without it, image formation would be impossible. But if the transition in the refractive index is sharp, reflections might be produced which can impair image quality. Moreover, the bright glare from an animal's eye can alert predators. Therefore, some insects have evolved fascinating nanoscale anti-reflection devices in the surface of their compound eyes.The compound eye of the Old Lady (Mormo maura) moth reveals highly ordered nano-structured conical protrusions on the corneal cuticle. It has been shown that this so-called ‘corneal nipple array’ or ‘moth-eye array’ has almost perfect anti-reflection properties. The crucial factor to reduce reflection of light is the nipple height. Only nipples with a height of > 50-200nm are anti-reflective. Corneal nipple arrays best known from nocturnal moths and butterflies (Lepidoptera), however they are widespread among insects and have probably evolved more than once. The nipples of the Old Lady moth are arranged in a hexagonal, almost crystalline fashion. In parallel to their anti-reflective function, the nipples also increase the surface area and thereby enhance the light sensitivity of the moth, though light transmittance increase may only be of minor importance compared to the potentially life-saving ability of the anti-reflective coating.Studying nano-structured corneal nipple arrays of insect eye facets helps to design better amorphous silicon thin film solar cells and improves the antireflective coating on notebooks and smartphones.
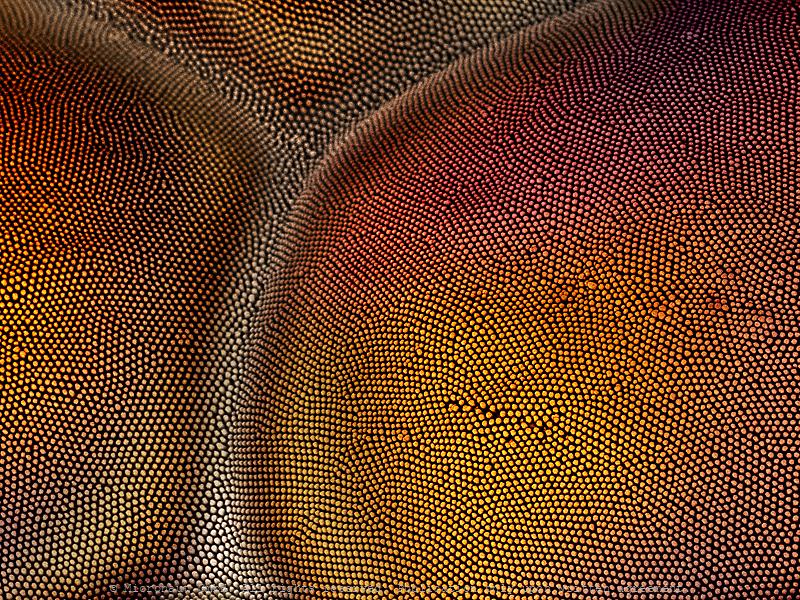
Anti-reflective coating, Nr.3
Antireflective Coating – ‘Corneal Nipple Arrays’ in Insect EyesEyes naturally possess a refracting surface - without it, image formation would be impossible. But if the transition in the refractive index is sharp, reflections might be produced which can impair image quality. Moreover, the bright glare from an animal's eye can alert predators. Therefore, some insects have evolved fascinating nanoscale anti-reflection devices in the surface of their compound eyes.The compound eye of the Old Lady (Mormo maura) moth reveals highly ordered nano-structured conical protrusions on the corneal cuticle. It has been shown that this so-called ‘corneal nipple array’ or ‘moth-eye array’ has almost perfect anti-reflection properties. The crucial factor to reduce reflection of light is the nipple height. Only nipples with a height of > 50-200nm are anti-reflective. Corneal nipple arrays best known from nocturnal moths and butterflies (Lepidoptera), however they are widespread among insects and have probably evolved more than once. The nipples of the Old Lady moth are arranged in a hexagonal, almost crystalline fashion. In parallel to their anti-reflective function, the nipples also increase the surface area and thereby enhance the light sensitivity of the moth, though light transmittance increase may only be of minor importance compared to the potentially life-saving ability of the anti-reflective coating.Studying nano-structured corneal nipple arrays of insect eye facets helps to design better amorphous silicon thin film solar cells and improves the antireflective coating on notebooks and smartphones.
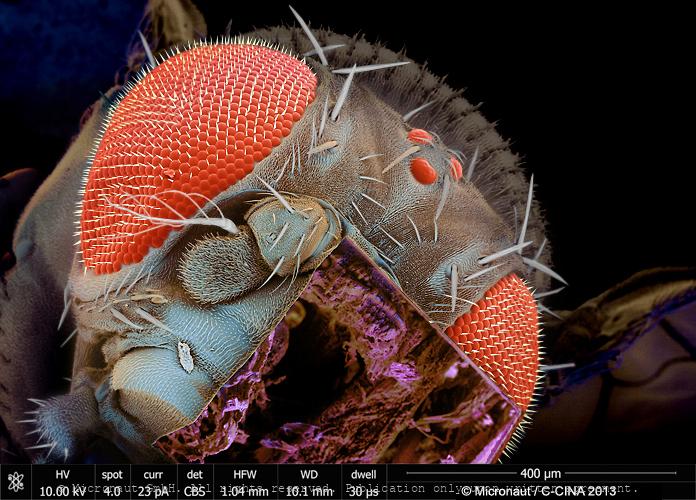
Fruitfly Head, FIB
Fruitfly head cut with Focused Ion Beam Microscope (FIB)
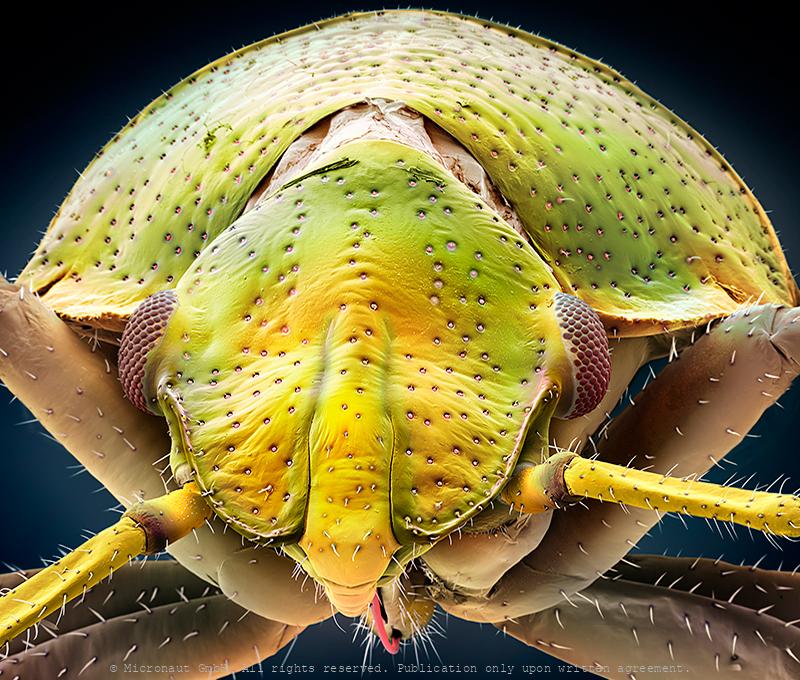
Juvenile eye (Pentatomidae)
An insect's compound eye is an engineering marvel: high resolution, wide field of view, and incredible sensitivity to motion, all in a compact package. Immature insects without metamorphosis, like this juvenile bug (Pentatomidae), are called nymphs. Examples are dragonflies, grasshoppers, or bugs. Nymphs do have compound eyes and look like adults, though more ommatidia (single lenses) are added each time the nymphs shed their skins. Each single lens does not see the entire picture. Instead, it only sees a small proportion and what the insect finally sees looks similar to a jigsaw puzzle. In general, compound eyes are fairly large in proportion to an insect's head. They are smallest in insects that spend their lives on the ground while flying insects have the largest compound eyes. Flying predatory insects have the very largest. A dragonfly's head is made up almost entirely of eyes and can see 360°. Each eye may have more than 20'000 single lenses (ommatidia). Male flying insects have larger eyes than females because they spend much of their time to flying around looking for females. It would be difficult to find mates if both sexes would search actively, so females usually stay still and let the males search for them. The most interesting insect eyes are those of whirlagig beetles (Family Gyrinidae). You've probably seen groups of these lively little black beetles on ponds and slow streams. They have two sets of compound eyes. One pair of eyes is on top of the head to see what's going on above, i.e. to watch for predators. The other pair of eyes is located below the water surface to look out for smaller aquatic insects on which they prey on themselves. Finally, insects to not necessarily see the same colors that we do. Many can see ultra-violet (UV) wavelenghts but do not see red (e.g. ants) at the other end of the spectrum. Other insects do not see yellow which is why yellow outside lights do not attract flying insects. Many flowers attract bees to pollinate
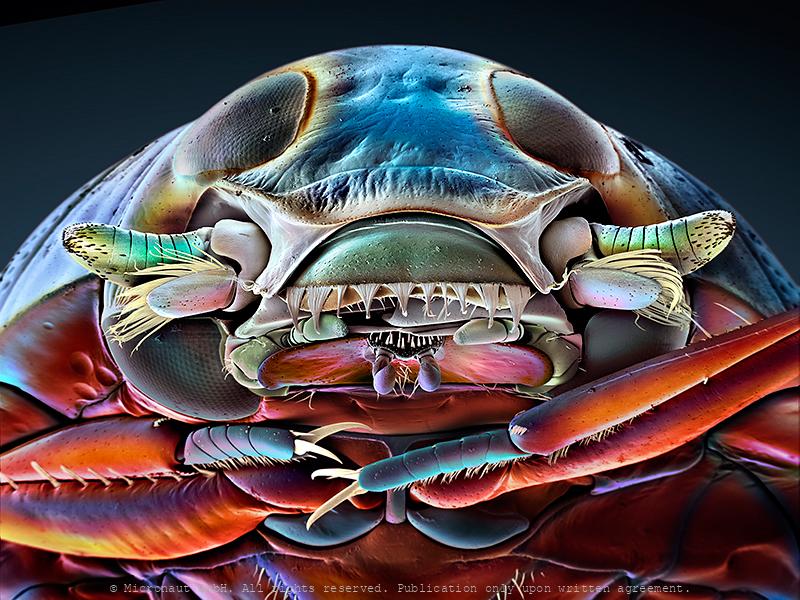
Divided Eye (Gyrinus substriatus)
Portrait of a Whirligig Beetle (Gyrinus substriatus). The most interesting insect compound eyes are those of whirligig beetles (Family Gyrinidae). You've probably seen groups of these lively little metallic beetles on ponds and slow streams. They have two sets of compound eyes. One pair of eyes is on top of the head to see what's going on above, i.e. to watch for predators. The other pair of eyes is located below the water surface to look out for smaller aquatic insects on which they prey on themselves. The antennae are also divided which is very unusual among beetles: the short and plump part is placed about at water level while the hairy lower part remains permanently submerged. The long bristles are used to detect vibrations of struggling insects that have fallen in and float with the current.
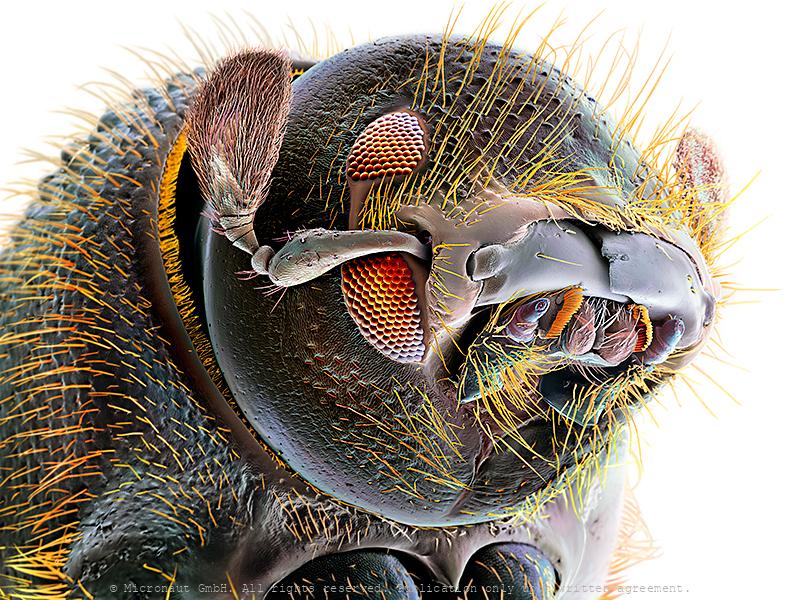
Timber Beetle (Trypodendron lineatum)
Portrait of a Bark Beetle (Spruce & Timber Beetle; Trypodendron lineatum). Bark beetles are a subfamily of the Snout beetles ('Weevils'). 154 species exist within Europe and between 4'000-5'000 worldwide. As primary destruents, they play an essential role in forrest ecosystems, living on dead wood or on dying trees. However, other species are known as notorious pests and are attacking (also) healthy trees. Trypodendron lineatum has a divided compound eye and is a monogamous species and shows brood care. The parents for example clean
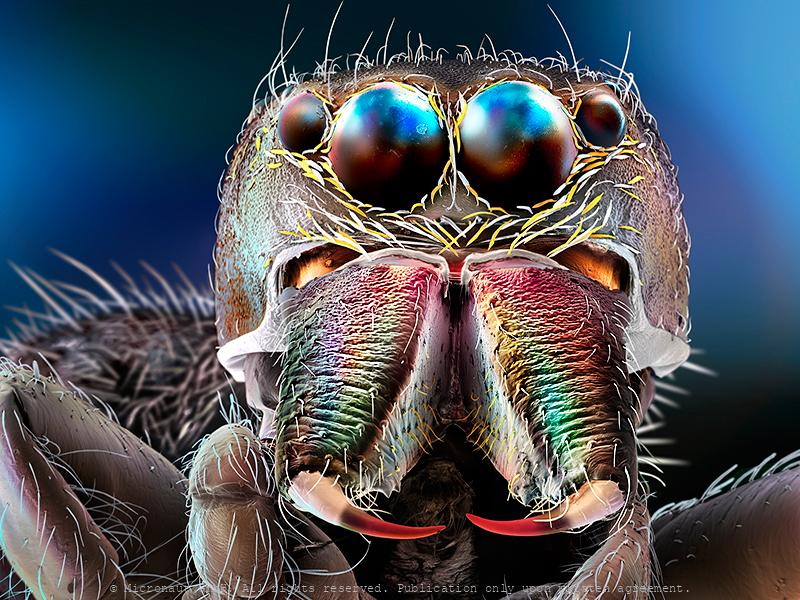
Jumping Spider
Often fuzzy and measuring less than a half inch in body length, Jumping spiders (Salticids) can run, climb, and - jump! Thereby the small predators can achieve stunning distances of more than 50 times its body size. Surprisingly, the extreme jumps are not the result of very strong or muscular legs. Instead, Jumping spiders quickly increase the blood pressure to their legs, which causes the legs to extend and propel the spider through the air. Prior to jumping, whatsoever, the spider glues silk threads to the surface beneath, to allow a quick and safe return to the perch, if required. Interestingly, Salticids rely on an excellent visual system. Like most other spiders, they have eight eyes on their face. An enormous pair in the center facing towards the front, and one adjacent pair of eyes to both sides give them an almost alien appearance. The remaining smaller eyes are located on the dorsal surface of the cephalothorax and allow almost 360° angle panoramic view. In general, animals have developed several different visual systems to accurately and reliably judge distance and depth. Humans, for example, have binocular stereovision. Because our eyes are spaced apart, they receive visual information from different angles, which our brains use to automatically triangulate distances. Other animals, such as insects, adjust the focal length of the lenses in their eyes, or move their heads side to side to create an effect called motion parallax — nearer objects will move across their field of vision more quickly than objects farther away. However, Jumping spiders have eyes that are too close together for binocular stereovision and don’t appear to use motion parallax while hunting. So how are these creatures able to perceive depth? In fact, these amazing creatures have developed a way of gauging distances that appears to be unique in the animal kingdom: rather than having a single layer of photoreceptor cells, as in our case, the spider's retina of the principal e
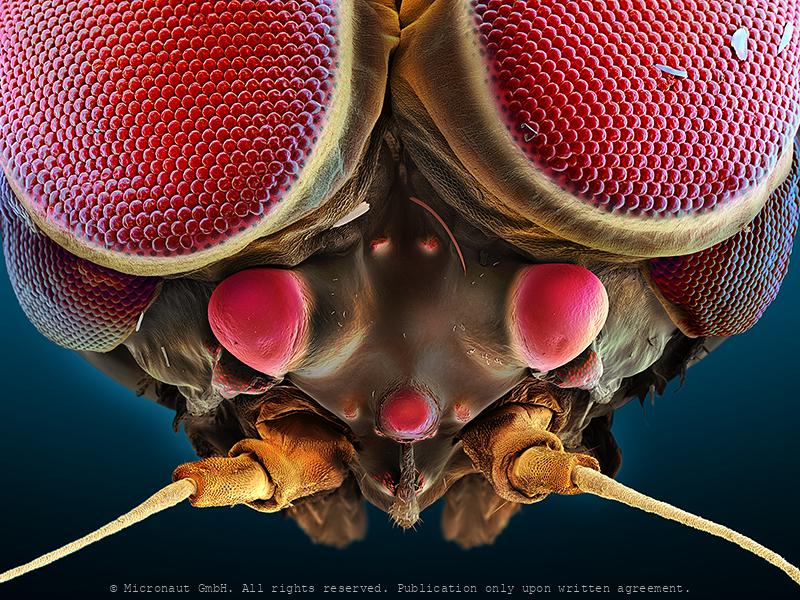
Turban Eye (Ephemeroptera)
Mayflies produce eggs that are laid on the surface of lakes or streams where they sink to the bottom. Naiads moult 20-30 times over a period of few months up to one year or even more. They live primarily on algae or diatoms, but some species are also predatory. The lifespan of adult mayflies is very short and varies between hours and 2-3 weeks. Since the primary function of the adult is reproduction, the mouthparts are non-functional and the digestive tract is filled with air. Adults have short flexible antennae, usually three ocelli and relatively large compound eyes. In most species, males additionally feature very large turban-shaped dorsal eyes, i.e. greatly enlarged compound eyes that are located on top of the head. The dorsal turban-eyes are especially designed to boost light sensitivity and allow vision at low light. At dawn, male mayflies fly close to the water surface in search of females, trying to detect females against the dim background of the sky. The turban-eyes are only capable of detecting ultra-violet (UV) light. In some species (e.g. European Mayfly) the turban-eye of the male is divided: The upper portion is for seeing movement, and the lower portion is specialized for seeing details. The females have smaller eyes.
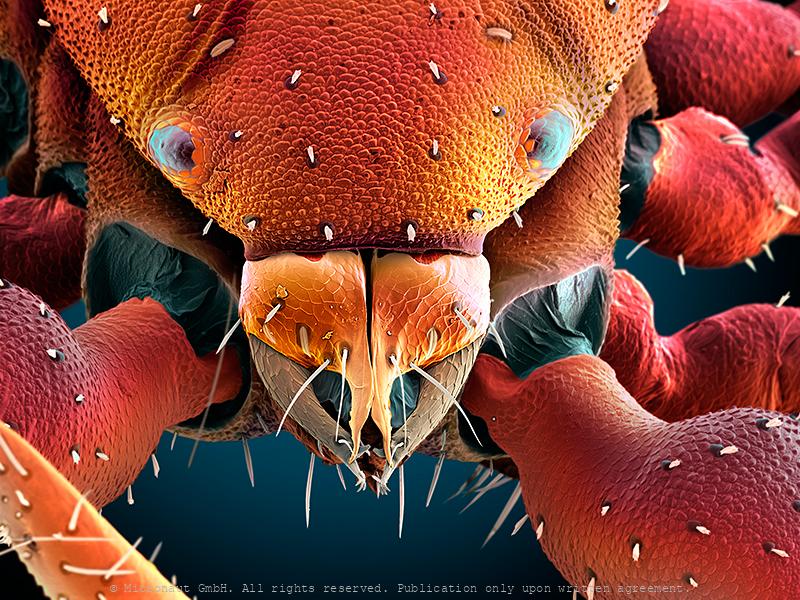
Pseudoscorpion (Chelifer cancroides), Nr.1
Pseudoscorpions are small arachnids with a flat, segmented, pear-shaped body. Despite being harmless and inoffensive creatures, this individual was able to move very quickly to escape. Pseudoscorpions got their name from a pair of huge pincers (pedipalps), resembling those of scorpions. But unlike true Scorpions they have a rounded abdomen that does not extend into a segmented tail with a stinger. The small creatures usually range from 2 to 8 millimetres (0.08 to 0.31 in) in length, with yellowish to red or dark brown colors. Pseudoscorpions are generally beneficial to humans and prey on clothes moth larvae, carpet beetle larvae, booklice, ants, mites, and small flies. Chelifer cancroides is the most commonly found species in homes, where they are often observed in rooms with dusty books. A venom gland and duct are located in the mobile finger of the pincers. The venom is used to capture and immobilize prey. During digestion, a mildly corrosive fluid is poured over the prey to liquify the remains externally before they are ingested. They enter homes by "riding along" attached to insects (known as phoresy). The jaws (chelicera; have a look at the beautiful structure in the center) are used to spin silk which is used to make disk-shaped cocoons for mating, molting, or waiting out cold weather. A comb-like structure (serrula externa), which can be seen on the left side of the chelicera in a close-up picture, is used for grooming. In the center of the chelicera is a membrane called serrula interna. It helps to prevent food and fluids from escaping. Also, two pairs of sensory setae can be found on the chelicera and additional structures which resemble plant pores are located on the cephalothorax (above the eyes in the overview picture). Scientists believe that the latter detect bending and stress on the cuticule and call them lyrifissures. Pseudoscorpions breathe through spiracles on their abdomen (not shown), a trait they share with the insects. Most species have o
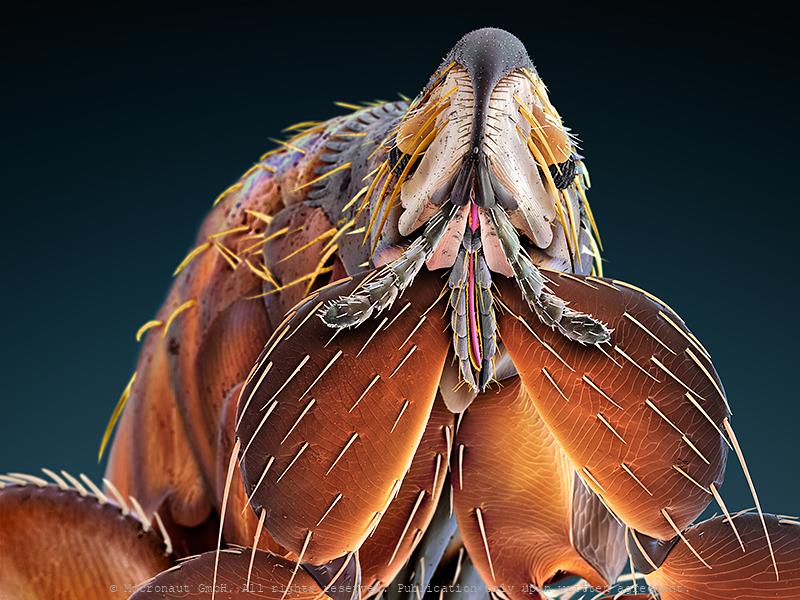
Bat flea (ev. Ischnopsyllus sp.)
Coloured scanning electron micrograph (SEM) of a Bat flea, showing its head, mandibles and powerful front legs. This parasite was found on a Daubenton's bat (Myotis daubentonii). The mandibles are used to pierce the host's skin and suck blood. As a result of living on nocturnal hosts Bat fleas have no eyes. Interestingly, Bat fleas have relatively short proboscis, measuring less than 200 µm. This is 33% less compared to the Cat flea (Ctenocephalides felis), 50% less compared to the common Rat flea (Xenopsylla cheopis), and even 90% less compared to the common European Mosquito (Culex pipiens). It remains to be determined if insects that parasitize bats evolved a reduced lenght of their blood sucking instruments due to the thin bat wing membranes that are well supplied with blood vessels and lack subcutaneous fat storage or dense fur. For the author the tiny animal reveals the grace of a flamenco dancer and provides a text-book example for the fascination of invisibly small creatures - and especially for those with a notoriously bad reputation generated in childhood from hearsay and fairy tales. Für den Autor hatte der Floh bereits auf der schwarz-weiss Aufnahme die Grazie einer Flamenco-Tänzerin ausgestrahlt. Nun erkennt man dank den Farben noch einiges mehr und ist gleichzeitig erstaunt wie perfekt die einzelnen Strukturen ineinander passen. Augen hat er keine, er lebt meist in völliger Dunkelheit, dafür sind die Fühler und tastenden Mundwerkzeuge prominent entwickelt und das ganze Gesicht ist äusserst fein behaart. Wenn man nichts sieht und auch kein Echolot hat, dann hilft eben der Tastsinn weiter - wir machen es genauso, falls das Licht einmal nicht funktioniert!
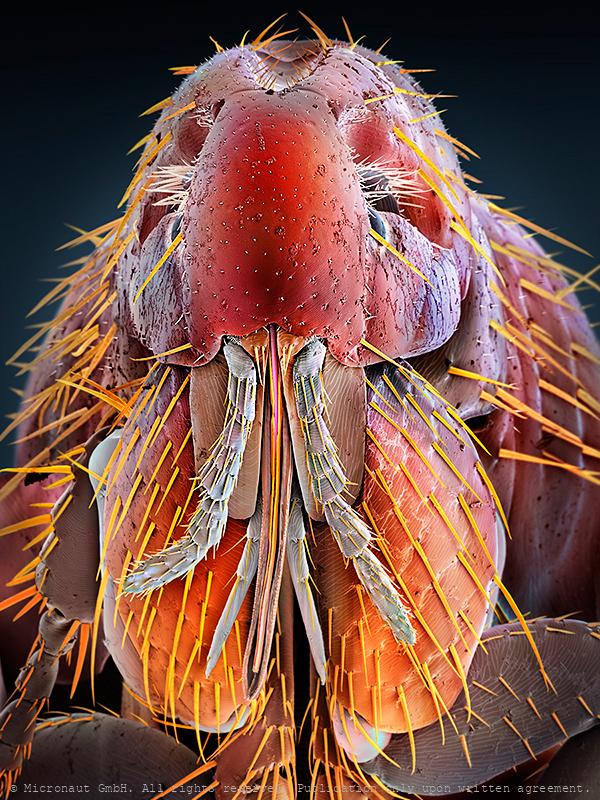
Rat flea (Xenopsylla cheopis)
In general, fleas belong to the insect order Siphonaptera. Their color varies between pale yellow and black and they are rather smalle, usually between 1-8mm long. The heavily sclerotized (armored) body is shiny. The rat flea's primary host is the rat (Rattus norvegicus), but the Oriental rat flea is best known as the vector for the bacteria Yersinia pestis from rats to humans, and for spreading the resulting plague (black death) across Asia and Europe in the middle ages. In the 14th century (between 1347 and 1352), the disease killed 25 million in Europe and between 75 to 100 million worldwide, within five years. Today, the plague is still not wiped out completely and the WHO registers annually between 1'000-3'000 cases worldwide. Like all fleas, Xenopsylla cheopis has mouthparts adapted to cutting through skin and sucking up blood that has pooled. In feeding, it secretes saliva into the wound to prevent the blood from coagulating. Along with the saliva, the flea secretes any bacteria it may have picked up by eating the blood of an infected individual into the host. When Yersinia pestis pathogens enter the gut of the flea, they multiply quickly, blocking food from entering the digestive system. This triggers the hungry flea to bite a new host, further spreading the bacteria. Today the Oriental rat flea can be found in temperate climates, but more often inhabits warm, tropical and subtropical regions, since it needs warm temperatures to pupate. It uses many different mammals as hosts, including rats and humans, and is known also to carry the murine typhus pathogen (Rickettsia typhi) and the mouse and rat tapeworms (Hymenolepis diminut and Hymenolepis nana), the latter can be transmitted when fleas are swallowed by pets or humans.
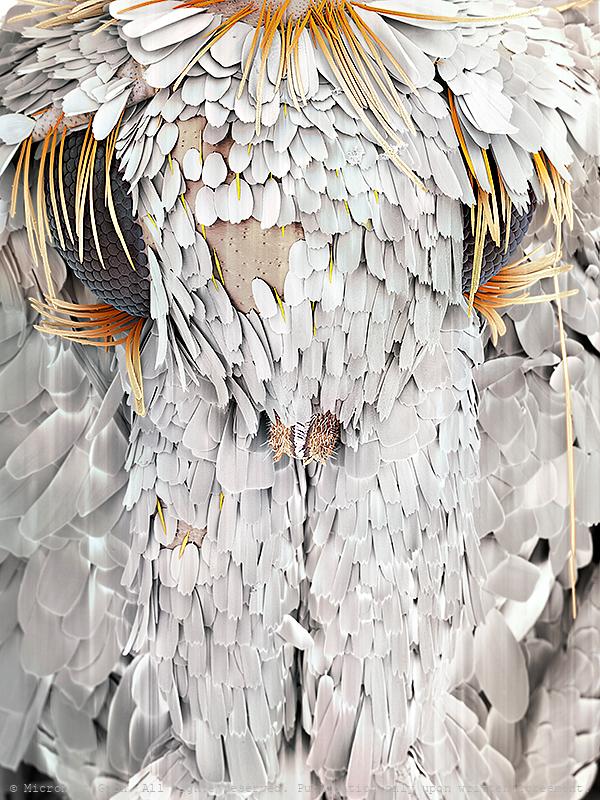
Moth head
Understanding other animals’ sensory abilities allows us to put our capabilities and limitations into perspective and lets us appreciate what we have. It also lets us imagine what it would be like to perceive the world through another creature’s eyes and - inspires technological innovations (ie: when designing robots or night vision goggles). In general the insect compound eye is made up of many separate lenses, called ommatidia. Moths have huge eyes covering almost their entire head. The huge compound eye even lets them see 'in the back of their head' at the cost of a relatively low resolution, because it depends from the number of photoreceptors (i.e. number of lenses) that can be grouped on the relatively small head. Thereby insects perceive the environment as 'patchwork image', as if we would look through the grid of a cellar window. Nevertheless the small moth has a few tricks up their sleeves for a better vision in the dark: (1) Neural summation: moths' brains store up images over time, giving their photoreceptors more chances to see in dim light (our photoreceptors 'stream' images in a constant flow which means old images are almost immediately lost). (2) Ultraviolet photoreceptor type. Instead of red, moths have ultraviolet photoreceoptor types helping them to find flowers at night: in contrast to humans, moths rely on color vision even at very low light without having to switch to achromatic vision to differentiate colors at low intensities. It may give them a less accurate sense of color at daylight, but moths see color at much lower intensities than humans. (3) Antireflective coatings: the ommatidia of moths' are covered with micro-structures which increases light efficiency at night and helps to avoid predators at daylight (frogs, lizards, birds). Anti-reflective coatings have become a key research area in solar panel construction, because they increase the efficiency.
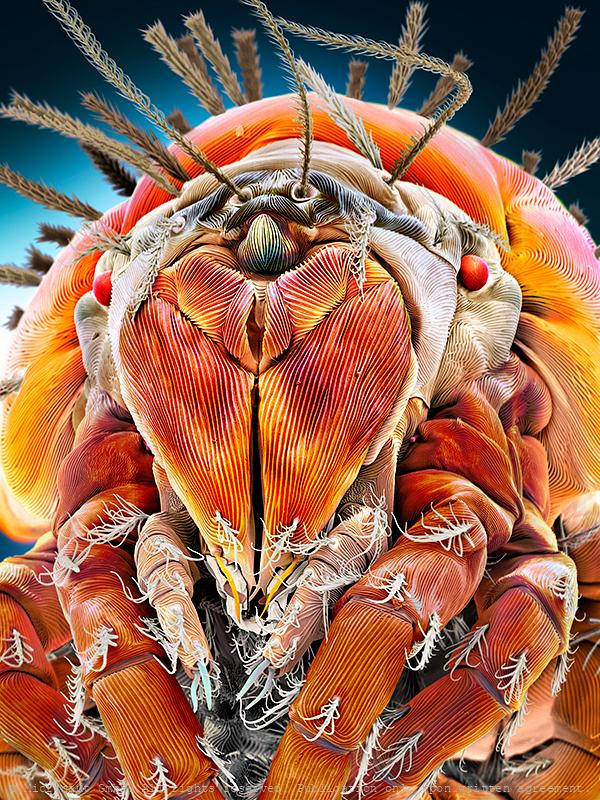
Mite (Speleorchestes sp.)
Mite of the genus Speleorchestes. The genus belongs to the enigmatic Endeostigmata – a perhaps paraphyletic basket that includes the most primitive-looking acariform mites. Endeostigmatans are found throughout the World, but are especially prominent in very dry habitats or dry microhabitats within more mesic ones. Both hot and cold deserts have more than their share of endeostigmatans. This group of mites includes some extraordinarily elongated worm-like species that can be found in the sands of Brazil beaches. However, this particular species is rather small and more mite-like, reaching about a quarter of a millimetre in lenght. Members of the Nanorchestidae also feed on fluids and so their foods are mysterious, although there is one report of a species munching on algae.
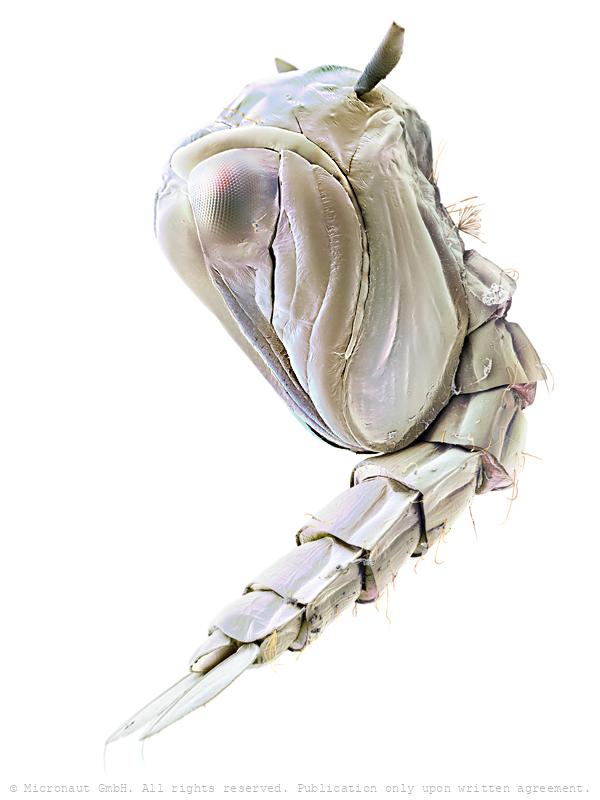
Pupae of malaria carrying mosquito (Anopheles gambiae)
Mosquito pupae (Anopheles gambiae): Head and thorax are merged into a cephalothorax, with the abdomen curving around underneath. The pupa is comma-shaped and can swim actively by flipping its abdomen, and it is commonly called a "tumbler" because of its swimming action. Mosquitos go through four stages of their life cycle: as with the larva, the pupa of most species must come to the surface frequently to breathe, which they do through a pair of respiratory trumpets on their cephalothoraces. In the course of the metamorphosis, the larva’s eye is subject to drastical differentiation and structural change. While the larva is spending most of its time feeding on algae, bacteria, and other microbes in the surface microlayer and only possess a pit-eye ocellus, the compound eyes of the adult are distinctly separated from one another and much more developed. Similar to other flying insect species, they are the dominant sensory organs for flight orientation.
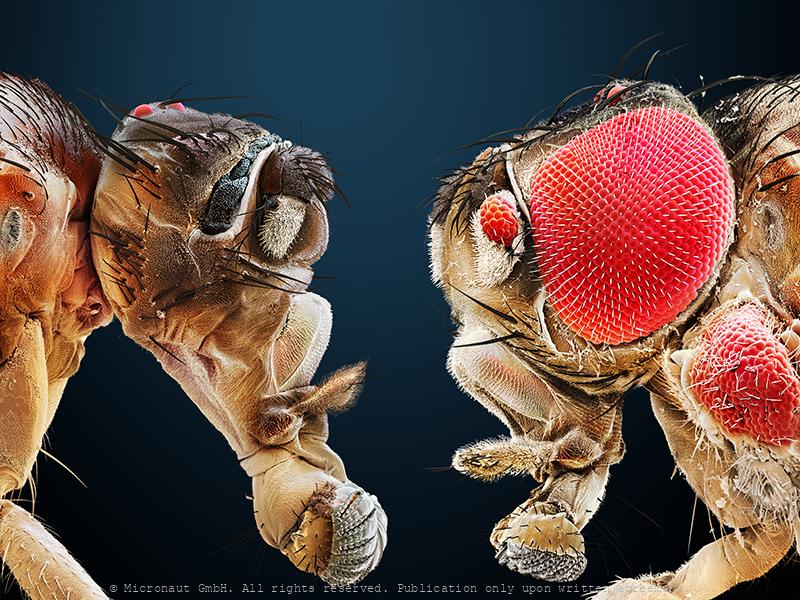
Uneven brothers (D. melanogaster), Nr.3
Coloured scanning electron micrograph (SEM) of two mutant Fruitfly (Drosophila melanogaster) heads. Wildtype (i.e. normal) Fruitflies have two compound eyes (red) - one on either side of the head. Small bristles between the single lenses of the eye make sure it cannot be covered with dust or dirt particles. Genetically manipulated Flies can either lack compound eyes completely (left) or have additional eyes on the antennae, legs and other body areas (right). Fruit flies are widely used in genetic experiments, particularly in mutation experiments, because they reproduce rapidly and their genetic systems are well understood. This image visualizes how easily the results of the genetic modification can be observed in the Fruitfly which is one of the main reasons why it is still the most frequently used model organism in genetics despite more than 100 years of experimental research. left: Sine oculis-1 (so1) variant which lacks the compound eyes completely. right: Eyeless variant with ectopically expressed compound eyes under dpp-promotor in all imaginal discs. Handkoloriertes Raster-Elektronen-Mikroskopiebild zweier Fruchtfliegen-Mutanten. Die Taufliege oder Fruchtfliege (Drosophila melanogaster) ist das klassische Untersuchungsobjekt in der Genetik und Entwicklungsbiologie. Wildtypen, d.h. genetisch unveränderte Fruchtfliegen, besitzen zwei grosse rote Komplexaugen - je eines auf jeder Seite des Kopfes. Die Komplexaugen werden durch Borsten zwischen den winzigen Einzelaugen vor Verschmutzung und Staub geschützt. Die Entwicklung des Auges wird während der Embryonalentwicklung Larve durch bestimmte Gene gesteuert. Durch Veränderungen (Mutation) von Genen lassen sich z.B. Position oder Morphologie der Komplexaugen gezielte beeinflussen. Im Vergleich zu einem Säugetier ist die Fruchtfliege genetisch viel einfacher aufgebaut und weist lediglich vier Paar Chromosomen auf. Diese Tatsache hat, neben der raschen Generationsfolge und einfachen Zucht, wesentlich dazu
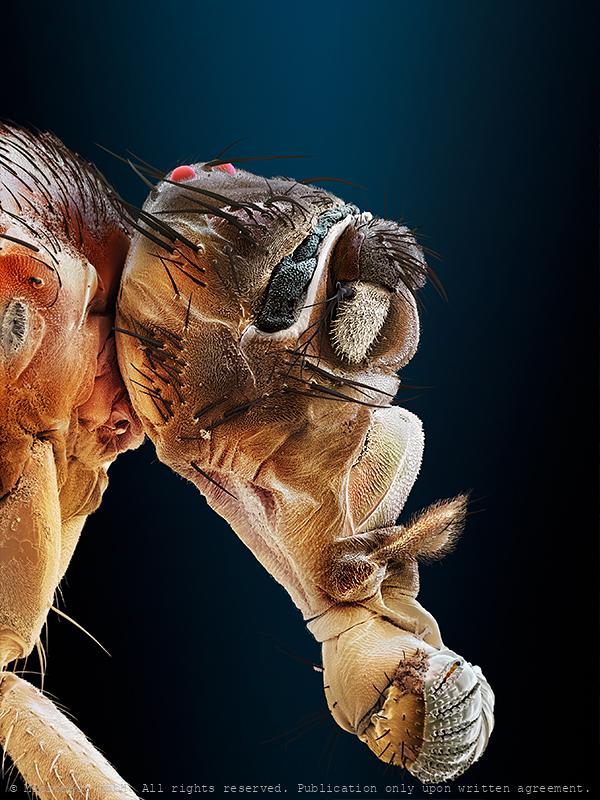
Uneven brothers (D. melanogaster), Nr.2
Coloured scanning electron micrograph (SEM) of two mutant Fruitfly (Drosophila melanogaster) heads. Wildtype (i.e. normal) Fruitflies have two compound eyes (red) - one on either side of the head. Small bristles between the single lenses of the eye make sure it cannot be covered with dust or dirt particles. Genetically manipulated Flies can either lack compound eyes completely (left) or have additional eyes on the antennae, legs and other body areas (right). Fruit flies are widely used in genetic experiments, particularly in mutation experiments, because they reproduce rapidly and their genetic systems are well understood. This image visualizes how easily the results of the genetic modification can be observed in the Fruitfly which is one of the main reasons why it is still the most frequently used model organism in genetics despite more than 100 years of experimental research. left: Sine oculis-1 (so1) variant which lacks the compound eyes completely. right: Eyeless variant with ectopically expressed compound eyes under dpp-promotor in all imaginal discs. Handkoloriertes Raster-Elektronen-Mikroskopiebild zweier Fruchtfliegen-Mutanten. Die Taufliege oder Fruchtfliege (Drosophila melanogaster) ist das klassische Untersuchungsobjekt in der Genetik und Entwicklungsbiologie. Wildtypen, d.h. genetisch unveränderte Fruchtfliegen, besitzen zwei grosse rote Komplexaugen - je eines auf jeder Seite des Kopfes. Die Komplexaugen werden durch Borsten zwischen den winzigen Einzelaugen vor Verschmutzung und Staub geschützt. Die Entwicklung des Auges wird während der Embryonalentwicklung Larve durch bestimmte Gene gesteuert. Durch Veränderungen (Mutation) von Genen lassen sich z.B. Position oder Morphologie der Komplexaugen gezielte beeinflussen. Im Vergleich zu einem Säugetier ist die Fruchtfliege genetisch viel einfacher aufgebaut und weist lediglich vier Paar Chromosomen auf. Diese Tatsache hat, neben der raschen Generationsfolge und einfachen Zucht, wesentlich dazu
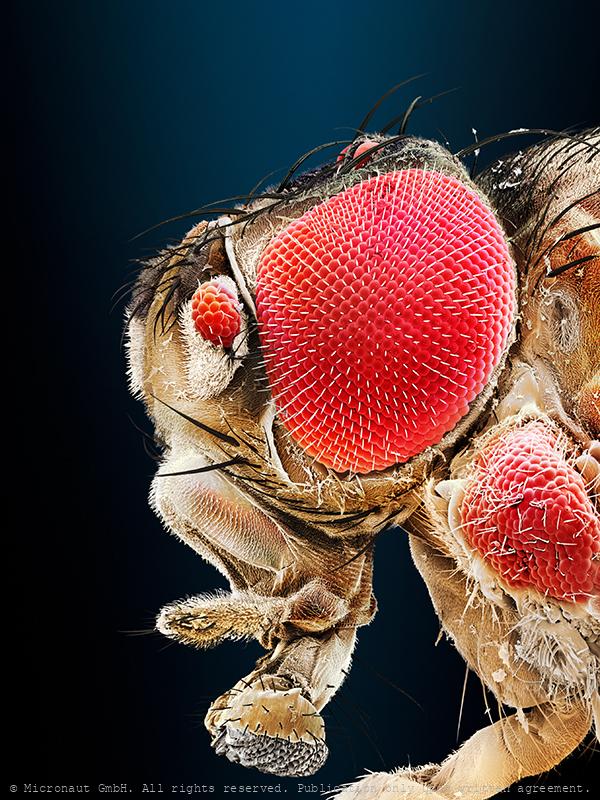
Uneven brothers (D. melanogaster), Nr.1
Coloured scanning electron micrograph (SEM) of two mutant Fruitfly (Drosophila melanogaster) heads. Wildtype (i.e. normal) Fruitflies have two compound eyes (red) - one on either side of the head. Small bristles between the single lenses of the eye make sure it cannot be covered with dust or dirt particles. Genetically manipulated Flies can either lack compound eyes completely (left) or have additional eyes on the antennae, legs and other body areas (right). Fruit flies are widely used in genetic experiments, particularly in mutation experiments, because they reproduce rapidly and their genetic systems are well understood. This image visualizes how easily the results of the genetic modification can be observed in the Fruitfly which is one of the main reasons why it is still the most frequently used model organism in genetics despite more than 100 years of experimental research. left: Sine oculis-1 (so1) variant which lacks the compound eyes completely. right: Eyeless variant with ectopically expressed compound eyes under dpp-promotor in all imaginal discs. Handkoloriertes Raster-Elektronen-Mikroskopiebild zweier Fruchtfliegen-Mutanten. Die Taufliege oder Fruchtfliege (Drosophila melanogaster) ist das klassische Untersuchungsobjekt in der Genetik und Entwicklungsbiologie. Wildtypen, d.h. genetisch unveränderte Fruchtfliegen, besitzen zwei grosse rote Komplexaugen - je eines auf jeder Seite des Kopfes. Die Komplexaugen werden durch Borsten zwischen den winzigen Einzelaugen vor Verschmutzung und Staub geschützt. Die Entwicklung des Auges wird während der Embryonalentwicklung Larve durch bestimmte Gene gesteuert. Durch Veränderungen (Mutation) von Genen lassen sich z.B. Position oder Morphologie der Komplexaugen gezielte beeinflussen. Im Vergleich zu einem Säugetier ist die Fruchtfliege genetisch viel einfacher aufgebaut und weist lediglich vier Paar Chromosomen auf. Diese Tatsache hat, neben der raschen Generationsfolge und einfachen Zucht, wesentlich dazu
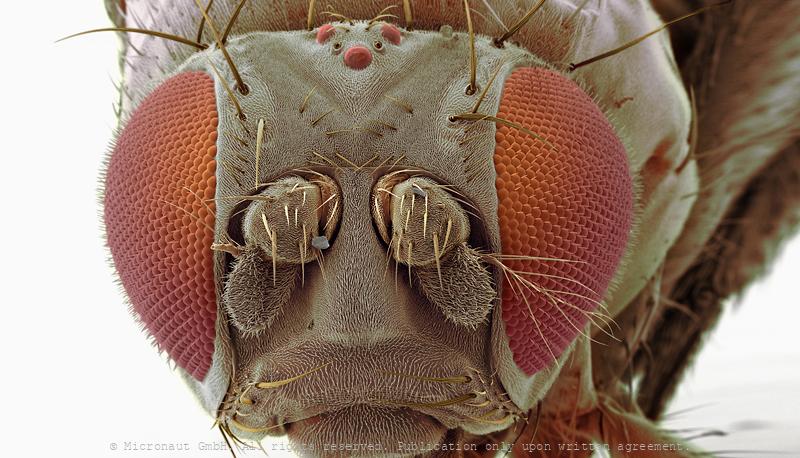
fly head
Fly head (Drosophila melanogaster)
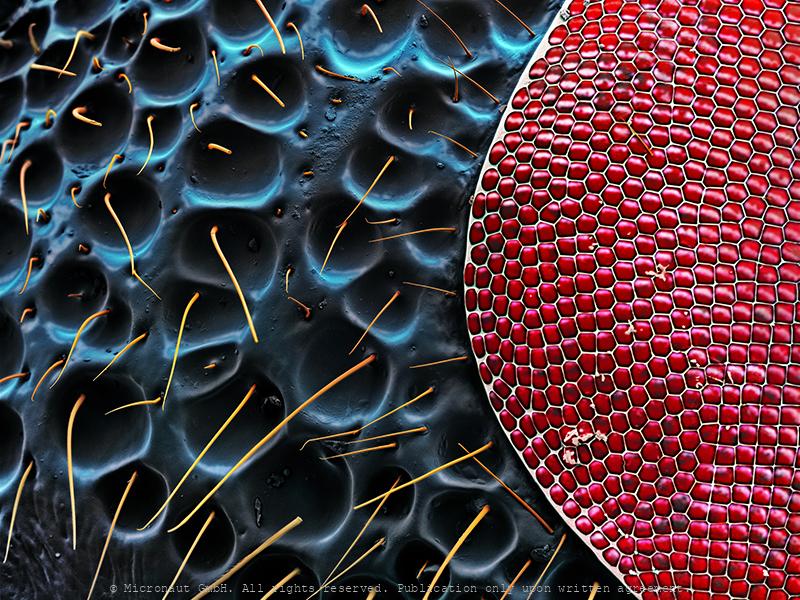
Wasp compound eye (Chrysis ignita)
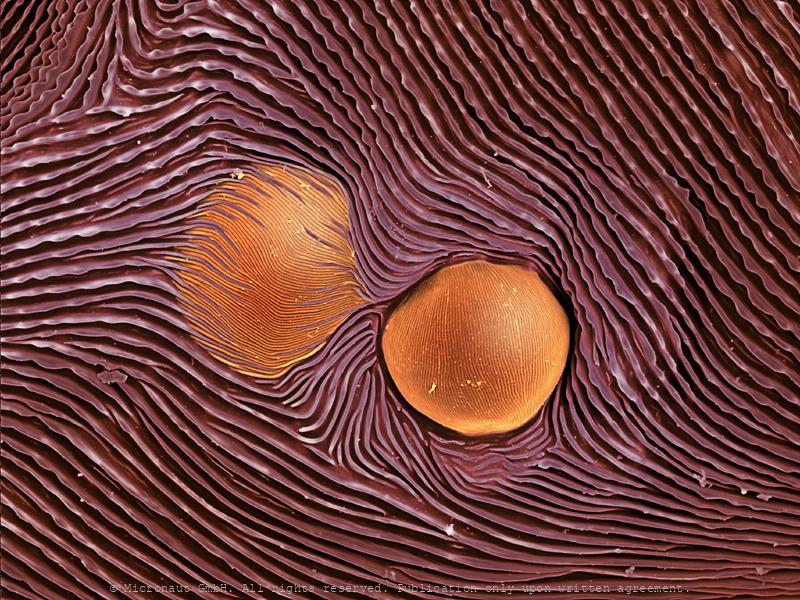
Spidermite eye
Most if not all Acari react to optical stimuli and thus demonstrate photosensitivity, yet not much is known about mite vision. Spider mites have two pairs of relatively large eyes. They can be used for color vision and to detect near-ultraviolet light waves.The pair of eyes on each side of Tetranychus urticae consists of fifteen retinular cells, six pigment cells, six corneal cells, and one vitreous cell. Responses of the summer form of adult females placed in near-ultraviolet and green light have shown that two separate photoreceptor systems are present and are able to discriminate between upper and lower leaf surface. Spider mites rely on vision to locate the preferred habitate, avoid deleterious UV-radiation, and to find a place to hide.
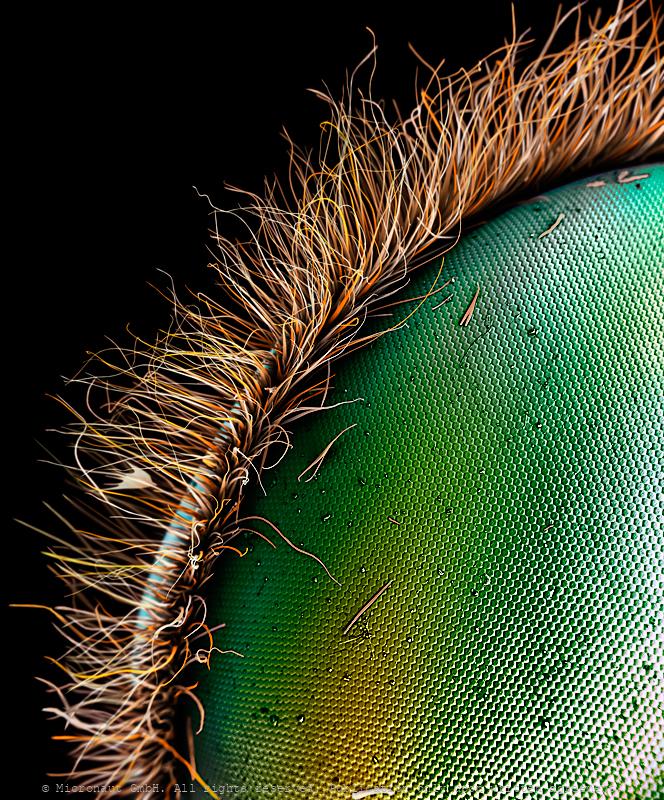
Hawkmoth eye (Acherontia atropos)
Compound eye of acherontia atropos
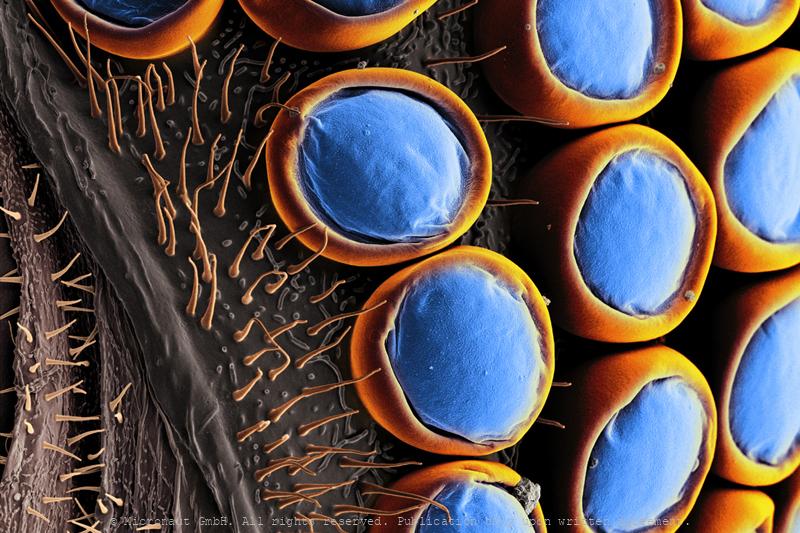
Midge compound eye structures
Midge compound eye structures
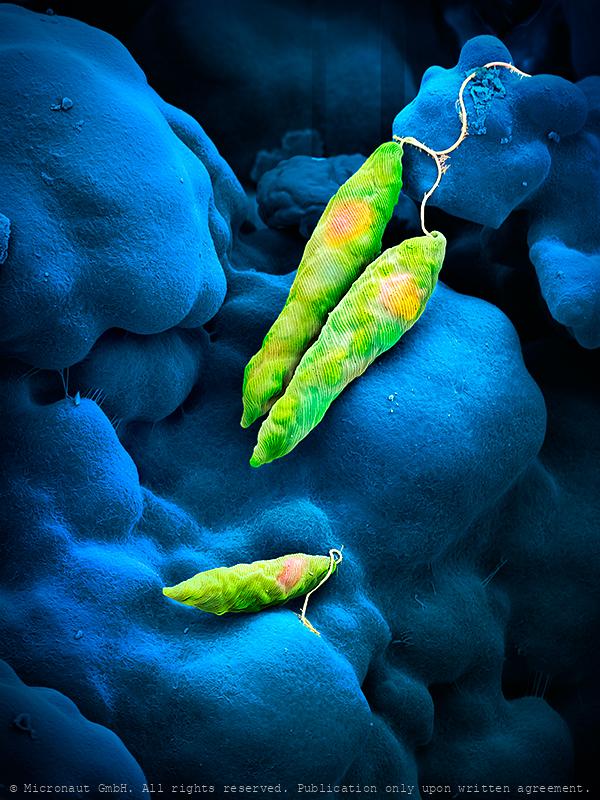
Flagellate (Euglena sp.)
Euglena is a genus of unicellular flagellate protists. It is the best known and most widely studied member of the phylum Euglenozoa, a diverse group containing some 44 genera and at least 800 species. Most species of Euglena have photosynthesizing chloroplasts within the body of the cell, which enable them to feed by autotrophy, like plants. However, they can also take nourishment heterotrophically, like animals. Euglena possess a red eyespot, an organelle composed of carotenoid pigment granules. The red spot itself is not thought to be photosensitive. Rather, it filters the sunlight that falls on a light-detecting structure at the base of the flagellum (a swelling, known as the paraflagellar body), allowing only certain wavelengths of light to reach it. As the cell rotates with respect to the light source, the eyespot partially blocks the source, permitting the Euglena to find the light and move toward it (a process known as phototaxis). Euglena lacks a cell wall. Instead, it has a pellicle made up of a protein layer supported by a substructure of microtubules, arranged in strips spiraling around the cell. The action of these pellicle strips sliding over one another gives Euglena its exceptional flexibility and contractility.
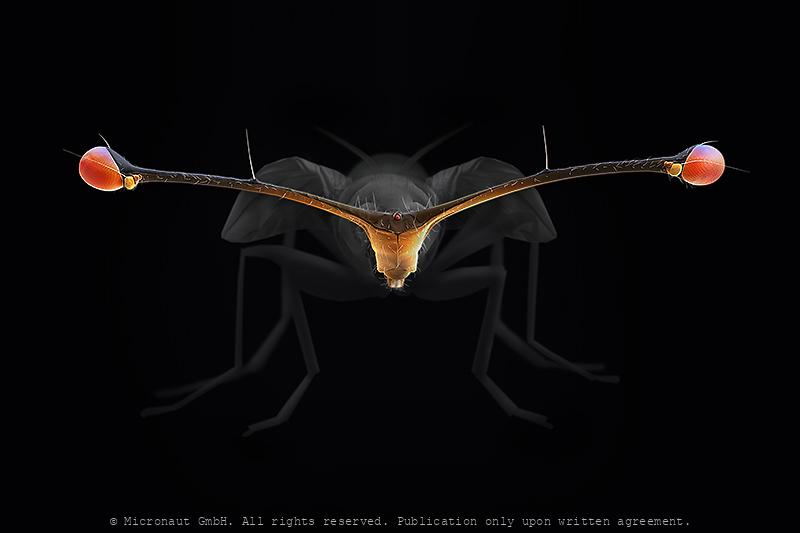
Stalk-Eyed Fly (Teleopsis whitei)
Among the true flies (Diptera) there are many examples of species with bizarre head projections, including eyes that are located on stalks. In the Diopsid family, the exaggeration of eye-stalks can be extreme, with males having an eyespan greater than their body length. Many stalk-eyed flies exhibit sexual dimorphism for eyespan, with males having much greater eyespan than females. Stalk-eyed flies are a well-documented example of sexual selection, because of the strong female preference for males with large eyespans.
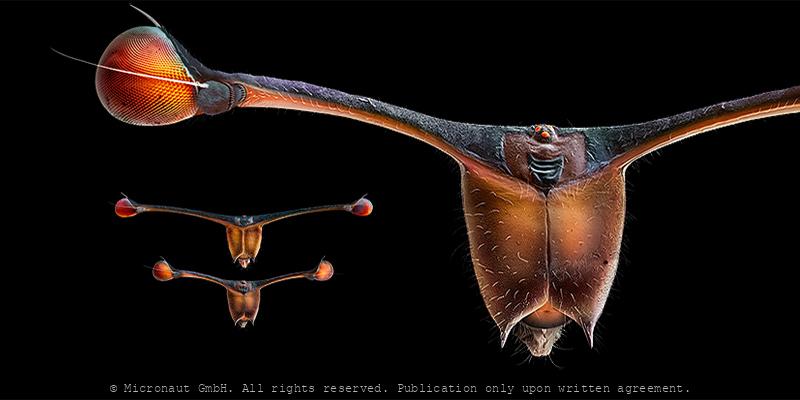
Stalk-Eyed Fly (Diasemopsis meigenii, female
Head of a stalk-eyed fly females and males. Stalk-eyed flies are distinguished by the possession of eyestalks: projections from the sides of the head with the eyes at the end. Males generally have more elongated eyestalks than females. This family (Diopsidae) of flies has been subject to a considerable amount of research due to their unique morphology to find out how it arises through forces of sexual selection and natural selection. Though the evolution of exaggerated male traits due to female mate choice was at one point considered rather controversial, stalk-eyed flies are now regarded as a classic example of animals that exhibit sexually selected traits.
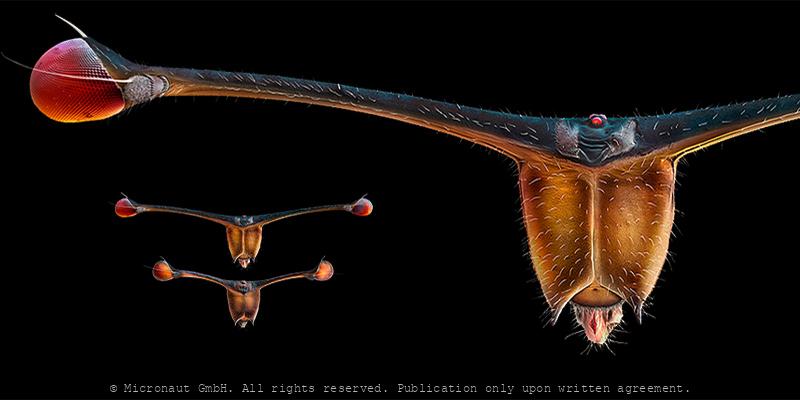
Stalk-Eyed Fly (Diasemopsis meigenii), male
Head of a stalk-eyed fly females and males. Stalk-eyed flies are distinguished by the possession of eyestalks: projections from the sides of the head with the eyes at the end. Males generally have more elongated eyestalks than females. This family (Diopsidae) of flies has been subject to a considerable amount of research due to their unique morphology to find out how it arises through forces of sexual selection and natural selection. Though the evolution of exaggerated male traits due to female mate choice was at one point considered rather controversial, stalk-eyed flies are now regarded as a classic example of animals that exhibit sexually selected traits.
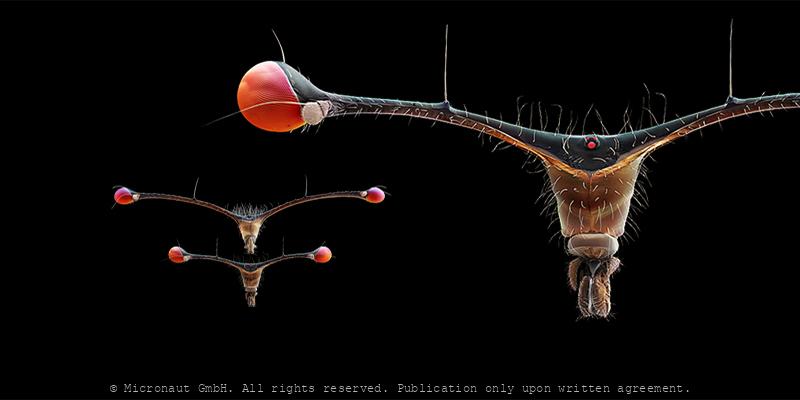
Stalk-Eyed Fly (C. thaii), female
Head of a stalk-eyed fly females and males. Stalk-eyed flies are distinguished by the possession of eyestalks: projections from the sides of the head with the eyes at the end. Males generally have more elongated eyestalks than females. This family (Diopsidae) of flies has been subject to a considerable amount of research due to their unique morphology to find out how it arises through forces of sexual selection and natural selection. Though the evolution of exaggerated male traits due to female mate choice was at one point considered rather controversial, stalk-eyed flies are now regarded as a classic example of animals that exhibit sexually selected traits.
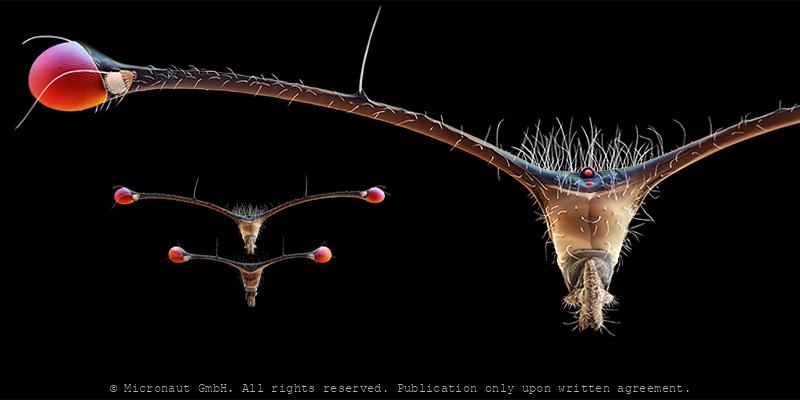
Stalk-Eyed Fly (C. thaii), male
Head of a stalk-eyed fly females and males. Stalk-eyed flies are distinguished by the possession of eyestalks: projections from the sides of the head with the eyes at the end. Males generally have more elongated eyestalks than females. This family (Diopsidae) of flies has been subject to a considerable amount of research due to their unique morphology to find out how it arises through forces of sexual selection and natural selection. Though the evolution of exaggerated male traits due to female mate choice was at one point considered rather controversial, stalk-eyed flies are now regarded as a classic example of animals that exhibit sexually selected traits.